Sickle cell retinopathy and systemic disease
Introduction
Background
In 1910, James B. Herrick described the first case of sickle cell disease (SCD) in a dental student from Grenada who presented with pulmonary symptoms (1). By 1949, Linus Pauling demonstrated differential migration of sickle cell hemoglobin (HbS) compared to normal hemoglobin (Hb) by gel electrophoresis (2). The sickle cell mutation was later described as a point mutation resulting in an amino acid substitution of glutamate to valine on the beta-globin subunit in Hb (3). As a result, deoxygenation in patients with SCD results in red blood cell sickling, and subsequent multiorgan system damage (4).
Worldwide, approximately 300,000 people affected by SCD are born each year (5). Over half of these patients are born in the Democratic Republic of the Congo, India, and Nigeria (5). This geographical bias is due to the sickle cell trait (heterozygous for the sickle cell mutation) providing a high degree of protection from severe malaria (6). In the United States, there are about 100,000 total patients with SCD (7). Due to migration and the slave trade, SCD is now present in most countries (5).
Rationale and knowledge gap
Unfortunately, patients with SCD experience significant morbidity and an average life expectancy 20 years less than the general population. In the United States, the life expectancy for patients with SCD is 54 years, while the life expectancy for patients without SCD is 76 years (8). Pediatric patients with SCD in sub-Saharan Africa have a high mortality rate, as 50% to 95% die by the age of 5 years (5). In recent years, exciting new treatment options have emerged to improve patients’ morbidity and mortality.
Objective
This review article aims to provide an overview of sickle disease for the ophthalmologist and to discuss emerging treatment options and current management of SCD ocular complications.
SCD overview
Genetics and pathophysiology
SCD is caused by a single alteration in the gene coding for the beta-globin subunit in Hb (3). Hb is a tetrameric molecule consisting of identical subunits (alpha, beta, gamma, or delta), each encoded by different genes (9). During fetal life, the predominant Hb is HbF (two alpha and two gamma subunits). In the postnatal period, HbF is normally replaced by HbA (two alpha and two beta subunits). HbS results from a single substitution of valine for glutamic acid in the beta globin subunit, and Hb C results from a single substitution of lysine for glutamic acid in the beta globin subunit. Patients with two HbS molecules (HbSS) have sickle cell anemia, and patients with the heterozygous mutation of hemoglobin SC (HbSC) have milder sickle cell anemia. Different genotypes result in different SCD phenotypes, with HbSS experiencing the most severe systemic variant and HbSC having a relatively better clinical course (10). However, the severity of retinopathy is reversed, as patients with HbSS have less severe retinopathy compared to those with HbSC (11). If just one HbS molecule is present, usually hemoglobin AS, the patient has sickle cell trait, which may have mild systemic symptoms and rarely demonstrates ophthalmic effects.
When deoxygenated, HbSS polymerizes and causes red blood cells to adopt a sickle shape (12). Sickled red blood cells have an increased adhesion to endothelial cells in blood vessel walls. Sickled cells can interact with platelets and white blood cells leading to vascular occlusion. Sickled cells hemolyze into free Hb, leading to endothelial cell dysfunction and inciting an inflammatory cascade (13). Chronic and recurrent sickling, hemolysis, and endovascular inflammation results in pain and organ damage (14). Vascular occlusion can lead to acute pain, acute chest syndrome, and avascular necrosis (15,16). Hemolysis and endothelial disruption can lead to ischemic stroke, leg ulcers, pulmonary hypertension, and priapism (15,16).
Diagnosis
Some countries, including the United States, screen all infants for SCD (16). Dried blood spot samples are screened through high-performance liquid chromatography or isoelectric testing to detect the presence of Hb variants (17). Solubility testing cannot differentiate between SCD or sickle cell trait, and may give false negative results (18). Diagnosis is confirmed by Hb electrophoresis (16). Despite screening, diagnosis and communication can be time consuming, requiring expensive equipment, skilled operators, and sometimes additional visits to explain the diagnosis (19). When not identified via screening, SCD might be diagnosed through evaluating a patient with normocytic anemia, atraumatic pain, or other systemic manifestations (16).
Systemic manifestations
Patients with SCD tend to experience both acute pain crises and chronic pain (16). Acute pain episodes are the most common complication of patients with SCD (20). Vital signs and laboratory values do not confirm or rule out pain crises (16). Severe pain crises are managed with analgesics, including opioids and nonsteroidal anti-inflammatory drugs (20,21). Unlike the general population, patients with chronic pain related to SCD are not more likely to become addicted to analgesics (16). Unless otherwise indicated, supplemental oxygen, intravenous fluids, and exchange transfusions are not necessary (20,21).
In children, splenic sequestration of sickled red blood cells is the most common cause of acute anemia, defined as a decline in baseline Hb of at least 2 g/dL (22). Due to hypovolemia and shock, emergent red blood cell transfusion is necessary (16). Since splenic sequestration can recur, splenectomy can be considered. Patients with SCD are also particularly susceptible to aplastic anemia, a condition in which parvovirus B19 infection results in reduced bone marrow production of red blood cells. Aplastic anemia should also be treated with emergent red blood cell transfusion (22).
Both pediatric and adult patients with SCD are at a higher risk of thromboembolism (23-25), and pharmacologic prophylaxis is recommended for all hospitalized patients with SCD (26). Acute treatment of thromboembolism is similar to the general population, although practitioners may have a lower threshold to institute chronic thromboembolism prophylaxis (26).
Male and female patients with SCD have diminished reproductive health (27). Pregnant patients with SCD have a higher rate of preeclampsia, urinary tract infections, low birth weight (28), and thromboembolic events (29). To mitigate the risk of thromboembolism, estrogen containing oral contraceptives should be avoided (30). In men, sperm abnormalities can occur in up to 90% of patients with SCD, possibly due to hypogonadism or testicular infarction (31,32).
Because of the increased risk of sepsis, stroke and perioperative complications, preventative guidelines have been put in place for patients with SCD. To mitigate sepsis, patients with SCD are recommended to receive penicillin prophylaxis daily until 5 years old and to receive appropriate immunizations (21). Patients with SCD aged 2–16 years old are recommended to receive annual transcranial Doppler ultrasounds (33). Abnormal transcranial Doppler ultrasound patients can receive monthly red blood cell (RBC) transfusions to decrease their risk of developing strokes (33). To reduce the risk of perioperative complications, especially acute chest syndrome (16), preoperative RBC transfusion can be considered in cases using general anesthesia that last greater than 1 hour (34).
Emerging treatments
For approximately 20 years, hydroxyurea was the only United States Food and Drug Administration (FDA) approved treatment for patients with SCD (14). Hydroxyurea is an oral medication that increases HbF production by inhibiting ribonucleotide reductase and also increases nitric oxide, decreases red blood cell adhesion, and decreases leukocytes. Hydroxyurea is considered the first-line therapy for most patients with SCD, and is prescribed as early as 9 months of age.
Crizanlizumab is an FDA approved oral antibody medication directed against P-selectin, an adhesion molecule implicated in endothelial cell vascular occlusion. A phase 2 trial demonstrated crizanlizumab decreases pain crises when compared to placebo in patients with SCD aged 16 to 65 years old (35). The authors recommend consideration of crizanlizumab as a second line treatment, in particular for patients with at least two pain crises per year on hydroxyurea or for patients intolerant to hydroxyurea.
L-glutamine is an FDA approved oral amino acid supplement for SCD that reduces reactive oxygen molecules to mitigate sickling and blood cell adhesion. A recent randomized controlled trial of 230 patients demonstrated a 25% reduction in acute pain crises compared to placebo, as well as significantly fewer hospitalizations (36). As with crizanlizumab, the authors recommend consideration of L-glutamine as a second line treatment for SCD pain management.
Voxelotor is an FDA approved oral medication that promotes HbS binding to oxygen, resulting in decreased sickling and hemolysis. In a recent phase 3 randomized clinical trial, voxelotor increased serum Hb by at least 1.0 g/dL (51%) compared to 7% of patients in the placebo group (37). Voxelotor is now a second line agent for sickle cell anemia.
In addition to these newly approved therapies, there are promising gene therapy experiments ongoing for SCD. One recently published case series by Kanter in 2022 used gene therapy with LentiGlobin infusions (bb1111; lovotibeglogene autotemcel) (38). Autologous transplantation of hematopoietic stem and progenitor cells transduced with a lentiviral vector encoding a modified beta-globin produced an anti-sickling manufactured Hb molecule (38). In their case series of 35 patients, modified autologous stem cell engraftment occurred in all patients. The anti-sickling manufactured Hb molecule represented at least 40% of total Hb, and serum Hb increased significantly from a median of 8.5 to 11 g/dL. Of the 25 patients experiencing vascular occlusive events, all had resolution over a median follow-up of 17 months. These 25 patients previously had a median 3.5 events per year in the 24 months prior to study enrollment. Other gene modification techniques are being developed, such as CRISP/Cas9 gene correction (39), pyruvate kinase activators (40), and proinflammatory cytokine inhibitors (41).
SCD ophthalmic manifestations
All ocular and orbital structures can be affected by ischemic events secondary to SCD (42), including orbital bone infarction (43), ischemic optic neuropathy (44), retinal artery occlusion (45), hyphema (46), secondary glaucoma (46), sickle cell maculopathy (47), and sickle cell retinopathy (48).
Orbital disease
Patients with SCD can develop infarctions of the orbital bones, commonly the greater wing of the sphenoid (49). Orbital infarction is more likely to occur in pediatric than adult patients due to their greater orbital bone marrow space (50). Orbital infarction may result in sterile inflammation and orbital hematoma. Symptoms of orbital bone infarction can include pain, headache, fever, periorbital edema, proptosis, and limited extraocular motility (49). Vision loss may occur if there is an exudative retinal detachment or optic nerve compression (51). Diagnosis is aided by computed tomography or magnetic resonance imaging, as well as doppler velocimetry to demonstrate arteriolar resistance (52,53). While rare, it is possible orbital bone infarctions are underdiagnosed given their tendency to spontaneously improve (42). Treatment consists of adequate hydration, pain control, steroids, and orbital decompression (52).
Hyphema and secondary glaucoma
Hyphema, ghost cells from vitreous hemorrhage (VH), or neovascularization (NV) of the angle can cause intraocular pressure (IOP) elevation and secondary glaucoma (46). Thus, all traumatic hyphema patients of African descent should be screened for a sickling hemoglobinopathy. Sickled red blood cells have a high tendency to occlude the trabecular meshwork, thus resulting in increased IOP. IOP lowering therapy should be initiated early, as the central retinal artery and optic disc may have impaired circulation and be more susceptible to damage at lower pressures than patients without SCD (44,45). When IOP lowering medication is insufficient, an early anterior chamber washout may prevent optic nerve damage (46). Carbonic anhydrase inhibitors (e.g., acetazolamide) should be used with extreme caution, as administration may worsen sickling through dehydration and metabolic acidosis (54).
Sickle cell retinopathy
Stages
Goldberg classified sickle cell retinopathy into five stages related to retinal vasculature alterations with disease progression (48). Stages I–II represent non-proliferative sickle cell retinopathy (NPSR), and Stages III–V represent proliferative sickle cell retinopathy (PSR). Peripheral arteriolar occlusions result in peripheral retinal nonperfusion (Stage I). Remodeling of the peripheral retinal vasculature causes arteriovenous (AV) anastomoses (Stage II). Transformation to Stage III represents development of NV at the posterior edge of the retinal nonperfusion (Figure 1A,1B). The classic white seafan occurs with autoinfarction of the NV, resulting in NV regression. Bleeding from the NV results in VH (Stage IV). Finally, degenerative changes in the vitreous over the leaking NV lead to the formation of preretinal tractional membranes that can ultimately cause a tractional retinal detachment (TRD) (Stage V).
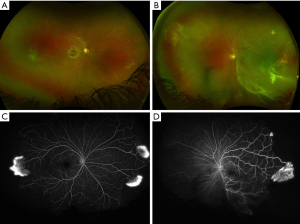
The Jamaican Cohort Study revealed the incidence of PSR is highest in patients with HbSC (43%) compared to HbSS (14%) by age 20.5 years (28). NPSR findings include retinal vascular abnormalities include retinal vascular occlusion, AV hairpin anastomoses, salmon-patch hemorrhages (intraretinal hemorrhages following artery occlusion), black sunbursts (focal areas of retinal pigment epithelium hypertrophy/hyperplasia), and iridescent spots (resorbed hemorrhages underneath the internal limiting membrane) (42). PSR is associated with macular occlusive disease, and the release of angiogenic mediators that promote NV, VH, and TRD (42).
Diagnosis
A thorough history, medical and ophthalmologic exam with dilated fundus exam can lead to clinical suspicion of SCD. Hb electrophoresis should be ordered to determine the SCD type. Ultra-widefield fluorescein angiography aids in the visualization of peripheral retinal vascular disease and is useful in diagnosing all forms of sickle cell retinopathy (55,56) (Figure 1C,1D). Optical coherence tomography and optical coherence tomography angiography can be helpful in the diagnosis of macular ischemia through the identification of macular thinning and flow voids in the superficial and deep retinal capillary plexi (57,58). Even in asymptomatic patients, identification of macular vascular changes might be helpful in detecting earlier disease, providing prognosis, and informing treatment decisions (42).
Management
All patients with sickle cell retinopathy should be co-managed by hematology and ophthalmology. As with other systemic diseases affecting the eye, treating the underlying disease often impacts the development and progression of sickle cell retinopathy. Here we will review local treatment options for ophthalmologists.
Laser photocoagulation
The average age of development of PSR is around 13 years of age (59), and screening for PSR is therefore recommended every 1 to 2 years from age 10 years (60). Ophthalmologists should consider scleral depressed exams, as retinal tears and rhegmatogenous retinal detachment (RRD) are more common in PSR than proliferative diabetic retinopathy (61). PSR may be treated with laser photocoagulation to the ischemic retina to cause regression of NV and decrease the risk of NV and TRD (62), but the benefit of laser remains controversial.
A Cochrane review assessed three randomized controlled trials of 414 eyes, each of which used a different laser technique, including focal scatter (63), feeder vessel coagulation (64), and sectoral scatter laser (65). No difference was reported in either complete regression of PSR (65) or the development of new PSR (64,65) when sectoral and feeder vessel coagulation was performed. Focal scatter laser reported regression in 55% of the treated group versus 28.6% of controls and progression of PSR in 10.5% of treated versus 25.7% of control eyes, but the review graded this to be low-certainty evidence. Both the studies using sectoral and feeder vessel coagulation reported greater vision loss in the control group compared to the treated group, while the focal scatter group showed no difference between the groups. Again, the certainty of this data was considered low. Lastly, all three studies showed less VH in the treated groups, but the data was again assessed to be low certainty.
Given these limited studies, it is not surprising that practice patterns regarding the use of laser photocoagulation for PSR vary. While many retina specialists implement laser as soon as PSR features are noted, others consider anti-vascular endothelial growth factor (VEGF) injections (66), which has been associated with regression of NV with PSR (67) and aiding operative management (68). Still others recommend observation (66), supported by the 40% autoinfarction rate of seafan NV complexes. More research is needed to further understand the risks and benefits of laser treatment and intravitreal injections of anti-VEGF compared to observation (62).
Vitreoretinal surgery
Vitreoretinal surgery can be performed for non-clearing VH, RRD, and TRD. Preoperative considerations are necessary, as patients with SCD are at higher risk of systemic and ocular vascular occlusive events (69). In 1971, Ryan and Goldberg reported anterior segment ischemia in 71% of patients receiving encircling scleral buckles (70). The authors proposed this high rate was due to compromised ciliary blood flow, inducing vascular occlusive disease. As a result, they recommended preoperative exchange transfusions and supplemental oxygen (70). Furthermore, Ryan and Goldberg recommended avoiding sympathomimetic agents, ciliary vessel damage, and extraocular muscle removal (70).
However, exchange transfusions have not been needed with more modern vitrectomy techniques, and scleral buckle placement seems to be well tolerated as long as the buckle indentation is relatively low-lying. A review of seven studies of 108 eyes undergoing posterior segment surgery reported no ischemic complications (69). Specifically, three larger recent case series from 2009–2018 reported no incidences of anterior segment ischemia despite not using exchange transfusions (71-73). Perhaps the increased use smaller gauge pars plana vitrectomy and shorter operating room times are reasons for reduced complications (69). Additionally, scleral buckles tend to be not as high and broad (69), and modern vitrectomy equipment has significantly improved IOP control with valved cannulas (73). In more complex vitreoretinal surgery cases, coordination of care between hematologists and ophthalmologists is still recommended to mitigate complications (69).
TRDs are exceedingly challenging to repair in SCD patients. The peripheral ischemic retina is thin and prone to iatrogenic retinal breaks, converting the case into a combined rhegmatogenous and TRD (72). Unlike diabetic patients, the traction points requiring dissection tend to be in the anterior retina, which is quite difficult in young phakic patients (72). Prevention of additional iatrogenic breaks may be aided by bimanual surgery with a chandelier, segmentation rather than delamination of preretinal membranes, and minimal tension on retinal surface.
Conclusions
SCD is a hemoglobinopathy that causes significant morbidity and mortality throughout the world. Patients with SCD experiencing deoxygenation develop red blood cell sickling with subsequent vascular occlusion and hemolysis. Vascular occlusion can cause acute pain, acute chest syndrome, and avascular necrosis, while hemolysis and endothelial disruption and cause ischemic stroke, leg ulcers, pulmonary hypertension, and priapism. Significant efforts have been made to reduce the risk of sepsis, stroke, and peri-operative complications including the use of hydroxyurea and the introduction of new FDA approved medications like crizanlizumab, L-gluatamine, and voxelotor. Additionally, several promising new gene therapy trials are ongoing. While PSR is a well described entity, guidelines regarding laser photocoagulation, intravitreal injections, and vitreoretinal surgery management remain based on expert advice and small case series due to limited published data and challenges performing randomized controlled trials. Further research is necessary to investigate optimal management strategies for patients with SCD.
Acknowledgments
Funding: None.
Footnote
Provenance and Peer Review: This article was commissioned by the Guest Editor (Kareem Moussa) for the series “The Retina and Systemic Disease” published in Annals of Eye Science. The article has undergone external peer review.
Peer Review File: Available at https://aes.amegroups.com/article/view/10.21037/aes-23-8/prf
Conflicts of Interest: The authors have completed the ICMJE uniform disclosure form (available at https://aes.amegroups.com/article/view/10.21037/aes-23-8/coif). The series “The Retina and Systemic Disease” was commissioned by the editorial office without any funding or sponsorship. D.A.B. is a consultant for Beyeonics and Gyroscope. B.K.W. is a consultant for Abbvie/Allergan, Alcon, Astellas/Iveric Bio, Castle Biosciences, Eyepoint Pharmaceuticals, Genentech, Immunocore, and Regeneron and has stock options with Lumata Health. The authors have no other conflicts of interest to declare.
Ethical Statement: The authors are accountable for all aspects of the work in ensuring that questions related to the accuracy or integrity of any part of the work are appropriately investigated and resolved. All clinical procedures described in this study were performed in accordance with the ethical standards of the institutional and/or national research committee(s) and with the Helsinki Declaration (as revised in 2013). Written informed consent was obtained from the patient for the publication of this article and accompanying images.
Open Access Statement: This is an Open Access article distributed in accordance with the Creative Commons Attribution-NonCommercial-NoDerivs 4.0 International License (CC BY-NC-ND 4.0), which permits the non-commercial replication and distribution of the article with the strict proviso that no changes or edits are made and the original work is properly cited (including links to both the formal publication through the relevant DOI and the license). See: https://creativecommons.org/licenses/by-nc-nd/4.0/.
References
- Herrick JB. Peculiar elongated and sickle-shaped red blood corpuscles in a case of severe anemia. 1910. Yale J Biol Med 2001;74:179-84. [PubMed]
- Pauling L, Itano HA. Sickle cell anemia a molecular disease. Science 1949;110:543-8. [Crossref] [PubMed]
- Ingram VM. Abnormal human haemoglobins. III. The chemical difference between normal and sickle cell haemoglobins. Biochim Biophys Acta 1959;36:402-11. [Crossref] [PubMed]
- Gaston M, Rosse WF. The cooperative study of sickle cell disease: review of study design and objectives. Am J Pediatr Hematol Oncol 1982;4:197-201. [PubMed]
- Piel FB, Hay SI, Gupta S, et al. Global burden of sickle cell anaemia in children under five, 2010-2050: modelling based on demographics, excess mortality, and interventions. PLoS Med 2013;10:e1001484. [Crossref] [PubMed]
- Piel FB, Patil AP, Howes RE, et al. Global distribution of the sickle cell gene and geographical confirmation of the malaria hypothesis. Nat Commun 2010;1:104. [Crossref] [PubMed]
- National Academies of Sciences, Engineering, and Medicine; Health and Medicine Division; Board on Population Health and Public Health Practice, et al. Addressing Sickle Cell Disease: A Strategic Plan and Blueprint for Action. Washington, DC, USA: National Academies Press; 2020.
- Lubeck D, Agodoa I, Bhakta N, et al. Estimated Life Expectancy and Income of Patients With Sickle Cell Disease Compared With Those Without Sickle Cell Disease. JAMA Netw Open 2019;2:e1915374. [Crossref] [PubMed]
- Stamatoyannopoulos G. Control of globin gene expression during development and erythroid differentiation. Exp Hematol 2005;33:259-71. [Crossref] [PubMed]
- Platt OS, Brambilla DJ, Rosse WF, et al. Mortality in sickle cell disease. Life expectancy and risk factors for early death. N Engl J Med 1994;330:1639-44. [Crossref] [PubMed]
- Downes SM, Hambleton IR, Chuang EL, et al. Incidence and natural history of proliferative sickle cell retinopathy: observations from a cohort study. Ophthalmology 2005;112:1869-75. [Crossref] [PubMed]
- Sundd P, Gladwin MT, Novelli EM. Pathophysiology of Sickle Cell Disease. Annu Rev Pathol 2019;14:263-92. [Crossref] [PubMed]
- Carden MA, Fasano RM, Meier ER. Not all red cells sickle the same: Contributions of the reticulocyte to disease pathology in sickle cell anemia. Blood Rev 2020;40:100637. [Crossref] [PubMed]
- Kato GJ, Piel FB, Reid CD, et al. Sickle cell disease. Nat Rev Dis Primers 2018;4:18010. [Crossref] [PubMed]
- Gladwin MT, Vichinsky E. Pulmonary complications of sickle cell disease. N Engl J Med 2008;359:2254-65. [Crossref] [PubMed]
- Kavanagh PL, Fasipe TA, Wun T. Sickle Cell Disease: A Review. JAMA 2022;328:57-68. [Crossref] [PubMed]
- Frömmel C. Newborn Screening for Sickle Cell Disease and Other Hemoglobinopathies: A Short Review on Classical Laboratory Methods-Isoelectric Focusing, HPLC, and Capillary Electrophoresis. Int J Neonatal Screen 2018;4:39. [Crossref] [PubMed]
- Association of Public Health Laboratories; Centers for Disease Control and Prevention. Hemoglobinopathies: Current Practices for Screening, Confirmation and Follow-up. 2015. Available online: https://stacks.cdc.gov/view/cdc/42387
- Kemper AR, Boyle CA, Brosco JP, et al. Ensuring the Life-Span Benefits of Newborn Screening. Pediatrics 2019;144:e20190904. [Crossref] [PubMed]
- Brandow AM, Carroll CP, Creary S, et al. American Society of Hematology 2020 guidelines for sickle cell disease: management of acute and chronic pain. Blood Adv 2020;4:2656-701. [Crossref] [PubMed]
- Thornburg CD, Files BA, Luo Z, et al. Impact of hydroxyurea on clinical events in the BABY HUG trial. Blood 2012;120:4304-10; quiz 4448. [Crossref] [PubMed]
- Ballas SK, Lieff S, Benjamin LJ, et al. Definitions of the phenotypic manifestations of sickle cell disease. Am J Hematol 2010;85:6-13. [Crossref] [PubMed]
- Brunson A, Lei A, Rosenberg AS, et al. Increased incidence of VTE in sickle cell disease patients: risk factors, recurrence and impact on mortality. Br J Haematol 2017;178:319-26. [Crossref] [PubMed]
- Brunson A, Keegan T, Mahajan A, et al. High incidence of venous thromboembolism recurrence in patients with sickle cell disease. Am J Hematol 2019;94:862-70. [Crossref] [PubMed]
- Kumar R, Stanek J, Creary S, et al. Prevalence and risk factors for venous thromboembolism in children with sickle cell disease: an administrative database study. Blood Adv 2018;2:285-91. [Crossref] [PubMed]
- Shet AS, Wun T. How I diagnose and treat venous thromboembolism in sickle cell disease. Blood 2018;132:1761-9. [Crossref] [PubMed]
- Boga C, Ozdogu H. Pregnancy and sickle cell disease: A review of the current literature. Crit Rev Oncol Hematol 2016;98:364-74. [Crossref] [PubMed]
- Lewis G, Thame M, Howitt C, et al. Pregnancy outcome in homozygous sickle cell disease: observations from the Jamaican Birth Cohort. BJOG 2021;128:1703-10. [Crossref] [PubMed]
- Boulet SL, Okoroh EM, Azonobi I, et al. Sickle cell disease in pregnancy: maternal complications in a Medicaid-enrolled population. Matern Child Health J 2013;17:200-7. [Crossref] [PubMed]
- Legardy JK, Curtis KM. Progestogen-only contraceptive use among women with sickle cell anemia: a systematic review. Contraception 2006;73:195-204. [Crossref] [PubMed]
- Ribeiro APMR, Silva CS, Zambrano JCC, et al. Compensated hypogonadism in men with sickle cell disease. Clin Endocrinol (Oxf) 2021;94:968-72. [Crossref] [PubMed]
- Taddesse A, Woldie IL, Khana P, et al. Hypogonadism in patients with sickle cell disease: central or peripheral? Acta Haematol 2012;128:65-8. [Crossref] [PubMed]
- Adams RJ, McKie VC, Hsu L, et al. Prevention of a first stroke by transfusions in children with sickle cell anemia and abnormal results on transcranial Doppler ultrasonography. N Engl J Med 1998;339:5-11. [Crossref] [PubMed]
- Wang WC, Ware RE, Miller ST, et al. Hydroxycarbamide in very young children with sickle-cell anaemia: a multicentre, randomised, controlled trial (BABY HUG). Lancet 2011;377:1663-72. [Crossref] [PubMed]
- Ataga KI, Kutlar A, Kanter J, et al. Crizanlizumab for the Prevention of Pain Crises in Sickle Cell Disease. N Engl J Med 2017;376:429-39. [Crossref] [PubMed]
- Niihara Y, Miller ST, Kanter J, et al. A Phase 3 Trial of l-Glutamine in Sickle Cell Disease. N Engl J Med 2018;379:226-35. [Crossref] [PubMed]
- Vichinsky E, Hoppe CC, Ataga KI, et al. A Phase 3 Randomized Trial of Voxelotor in Sickle Cell Disease. N Engl J Med 2019;381:509-19. [Crossref] [PubMed]
- Kanter J, Walters MC, Krishnamurti L, et al. Biologic and Clinical Efficacy of LentiGlobin for Sickle Cell Disease. N Engl J Med 2022;386:617-28. [Crossref] [PubMed]
- Frangoul H, Altshuler D, Cappellini MD, et al. CRISPR-Cas9 Gene Editing for Sickle Cell Disease and β-Thalassemia. N Engl J Med 2021;384:252-60. [Crossref] [PubMed]
- van Dijk MJ, Rab MAE, van Oirschot BA, et al. Safety and efficacy of mitapivat, an oral pyruvate kinase activator, in sickle cell disease: A phase 2, open-label study. Am J Hematol 2022;97:E226-9. [Crossref] [PubMed]
- Rees DC, Kilinc Y, Unal S, et al. A randomized, placebo-controlled, double-blind trial of canakinumab in children and young adults with sickle cell anemia. Blood 2022;139:2642-52. [Crossref] [PubMed]
- Abdalla Elsayed MEA, Mura M, Al Dhibi H, et al. Sickle cell retinopathy. A focused review. Graefes Arch Clin Exp Ophthalmol 2019;257:1353-64. [Crossref] [PubMed]
- Blank JP, Gill FM. Orbital infarction in sickle cell disease. Pediatrics 1981;67:879-81. [Crossref] [PubMed]
- Perlman JI, Forman S, Gonzalez ER. Retrobulbar ischemic optic neuropathy associated with sickle cell disease. J Neuroophthalmol 1994;14:45-8. [Crossref] [PubMed]
- Fine LC, Petrovic' V, Irvine AR, et al. Spontaneous central retinal artery occlusion in hemoglobin sickle cell disease. Am J Ophthalmol 2000;129:680-1. [Crossref] [PubMed]
- Goldberg MF. Sickled erythrocytes, hyphema, and secondary glaucoma: I. The diagnosis and treatment of sickled erythrocytes in human hyphemas. Ophthalmic Surg 1979;10:17-31. [PubMed]
- Dell'Arti L, Barteselli G, Riva L, et al. Sickle cell maculopathy: Identification of systemic risk factors, and microstructural analysis of individual retinal layers of the macula. PLoS One 2018;13:e0193582. [Crossref] [PubMed]
- Goldberg MF. Classification and pathogenesis of proliferative sickle retinopathy. Am J Ophthalmol 1971;71:649-65. [Crossref] [PubMed]
- Alsuhaibani AH, Marzouk MA. Recurrent infarction of sphenoid bone with subperiosteal collection in a child with sickle cell disease. Ophthalmic Plast Reconstr Surg 2011;27:e136-8. [Crossref] [PubMed]
- Graham R. Serjeant BES. Sickle Cell Disease. 3rd edition. Oxford University Press; 2001.
- Helen OO, Ajite KO, Oyelami OA, et al. Bilateral orbital infarction and retinal detachment in a previously undiagnosed sickle cell hemoglobinopathy African child. Niger Med J 2013;54:200-2. [Crossref] [PubMed]
- Brandow AM, Liem R. Sickle Cell Disease in the Emergency Department: Atypical Complications and Management Clin Pediatr Emerg Med 2011;12:202-12. [Crossref] [PubMed]
- Ferrão TO, Martins-Filho PR, Aragão C, et al. Doppler velocimetry of the orbital arteries in patients with sickle cell anemia: relationship with biomarkers of hemolysis. Radiol Bras 2017;50:103-8. [Crossref] [PubMed]
- Chen RW, Flynn HW, Fekrat S, et al. Sickle retinopathy. In: Scott IU, Regillo CD, Flynn HW, et al. editors. Vitreoretinal disease. Diagnosis management and clinical pearls. New York: Thieme Publishers; 2018.
- Tan CS, Chew MC, van Hemert J, et al. Measuring the precise area of peripheral retinal non-perfusion using ultra-widefield imaging and its correlation with the ischaemic index. Br J Ophthalmol 2016;100:235-9. [Crossref] [PubMed]
- Tsui I, Williams BK Jr, Kok YO, et al. Reliability of Ischemic Index Grading in Common Retinal Vascular Diseases. Ophthalmic Surg Lasers Imaging Retina 2015;46:618-25. [Crossref] [PubMed]
- Pahl DA, Green NS, Bhatia M, et al. Optical Coherence Tomography Angiography and Ultra-widefield Fluorescein Angiography for Early Detection of Adolescent Sickle Retinopathy. Am J Ophthalmol 2017;183:91-8. [Crossref] [PubMed]
- Sanfilippo CJ, Klufas MA, Sarraf D, et al. Optical Coherence Tomography Angiography of Sickle Cell Maculopathy. Retin Cases Brief Rep 2015;9:360-2. [Crossref] [PubMed]
- Rosenberg JB, Hutcheson KA. Pediatric sickle cell retinopathy: correlation with clinical factors. J AAPOS 2011;15:49-53. [Crossref] [PubMed]
- Yawn BP, Buchanan GR, Afenyi-Annan AN, et al. Management of sickle cell disease: summary of the 2014 evidence-based report by expert panel members. JAMA 2014;312:1033-48. [Crossref] [PubMed]
- McLeod DS, Merges C, Fukushima A, et al. Histopathologic features of neovascularization in sickle cell retinopathy. Am J Ophthalmol 1997;124:455-72. [Crossref] [PubMed]
- Myint KT, Sahoo S, Thein AW, et al. Laser therapy for retinopathy in sickle cell disease. Cochrane Database Syst Rev 2022;12:CD010790. [PubMed]
- Farber MD, Jampol LM, Fox P, et al. A randomized clinical trial of scatter photocoagulation of proliferative sickle cell retinopathy. Arch Ophthalmol 1991;109:363-7. [Crossref] [PubMed]
- Jampol LM, Condon P, Farber M, et al. A randomized clinical trial of feeder vessel photocoagulation of proliferative sickle cell retinopathy. I. Preliminary results. Ophthalmology 1983;90:540-5. [Crossref] [PubMed]
- Sayag D, Binaghi M, Souied EH, et al. Retinal photocoagulation for proliferative sickle cell retinopathy: a prospective clinical trial with new sea fan classification. Eur J Ophthalmol 2008;18:248-54. [Crossref] [PubMed]
- Mishra K, Bajaj R, Scott AW. Variable Practice Patterns for Management of Sickle Cell Retinopathy. Ophthalmol Retina 2021;5:715-7. [Crossref] [PubMed]
- Siqueira RC, Costa RA, Scott IU, et al. Intravitreal bevacizumab (Avastin) injection associated with regression of retinal neovascularization caused by sickle cell retinopathy. Acta Ophthalmol Scand 2006;84:834-5. [Crossref] [PubMed]
- Moshiri A, Ha NK, Ko FS, et al. Bevacizumab presurgical treatment for proliferative sickle-cell retinopathy-related retinal detachment. Retin Cases Brief Rep 2013;7:204-5. [Crossref] [PubMed]
- Nithianandan H, Sridhar J. Surgical and Medical Perioperative Management of Sickle Cell Retinopathy: A Literature Review. Int Ophthalmol Clin 2020;60:77-87. [Crossref] [PubMed]
- Ryan SJ, Goldberg MF. Anterior segment ischemia following scleral buckling in sickle cell hemoglobinopathy. Am J Ophthalmol 1971;72:35-50. [Crossref] [PubMed]
- Ho J, Grabowska A, Ugarte M, et al. A comparison of 23-gauge and 20-gauge vitrectomy for proliferative sickle cell retinopathy - clinical outcomes and surgical management. Eye (Lond) 2018;32:1449-54. [Crossref] [PubMed]
- Chen RW, Flynn HW Jr, Lee WH, et al. Vitreoretinal management and surgical outcomes in proliferative sickle retinopathy: a case series. Am J Ophthalmol 2014;157:870-875.e1. [Crossref] [PubMed]
- Williamson TH, Rajput R, Laidlaw DA, et al. Vitreoretinal management of the complications of sickle cell retinopathy by observation or pars plana vitrectomy. Eye (Lond) 2009;23:1314-20. [Crossref] [PubMed]
Cite this article as: Anibire OO, Brill DA, Williams BK Jr. Sickle cell retinopathy and systemic disease. Ann Eye Sci 2024;9:11.