A narrative review of limbal stem cell deficiency & severe ocular surface disease
Introduction
The corneal epithelium is a stratified squamous epithelium and must regenerate continuously. Clinically, this is understood intuitively; a straightforward corneal abrasion is easily identified and can be dependably expected to resolve in a matter of hours to days (depending on the size) in a healthy cornea. However, understanding the processes which govern this series of events has been and continues to be the subject of significant interest for corneal specialists. In Vogt’s original atlas of the cornea from 1921, he described radially oriented structures (termed palisades), which were located most prominently at the superior and inferior limbus (Figure 1). He found that they were located just peripheral to the terminal capillary loops of the conjunctiva and had distinct radially oriented arterial and venous components. The palisades were described to be white in color and to become more prominent with aging (1). 3H-thymidine labeling of the limbal epithelium further demonstrated that the corneal epithelium healed in a centripetal manner with cells originating from the limbus near these structures (2). The rate of movement has now been characterized with scanning microscopy, ranging from 1.7 to 32 µm a day (3). Further study in green fluorescent protein (GFP) expressing mice with in vivo microscopy has also demonstrated the centripetal movement, with an average of 26 µm a day (4). It is now understood that the palisades of Vogt (POV) are home to limbal stem cells which repopulate the epithelium. The maintenance of this microenvironment is critical to the regenerative potential of the cornea. These limbal stem cells move centripetally first, differentiating into the basal epithelial cells, proliferate, and then move anteriorly towards the surface to be shed.
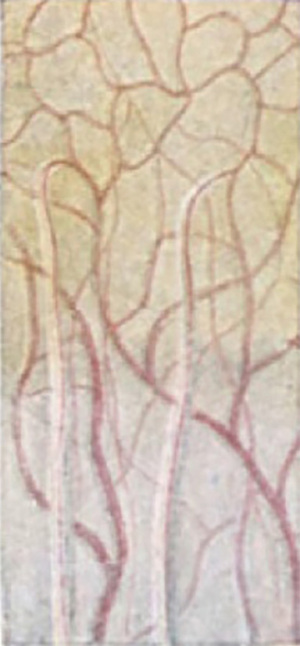
Limbal stem cell deficiency (LSCD) describes the clinical condition when there is dysfunction of the corneal epithelial stem/progenitor cells and the inability to sustain the normal homeostasis of the corneal epithelium (5). These cells can be damaged by a number of different processes as detailed below. The loss of limbal stem cells results in the replacement of corneal epithelial cells with conjunctival epithelial cells, decreasing the normal clarity of the cornea and leading to impaired healing, scarring and vision loss. Even with only a remainder of 7% of limbal stem cells, there is still the capacity for the corneal epithelium to be repopulated with modern surgical techniques (6). The limbus also acts as a barrier for conjunctival invasion onto the cornea. Partial LSCD occurs when there is incomplete conjunctivalization of the cornea whereas total LSCD (due to complete loss of corneal epithelial stem cells) leads to complete conjunctivalization of the cornea.
This review will focus on the clinical manifestations and causes of LSCD, including a summary of the 2019 global consensus published from the Limbal Stem Cell Working Group by the Cornea Society, as well as the advantages and limitations of current diagnostic methods. In addition, it highlights new epidemiologic data which has shown the burden of LSCD as well as provide an update regarding the pathophysiology, diagnosis, and management of some of the most common causes of LSCD. We present this article in accordance with the Narrative Review reporting checklist (available at https://aes.amegroups.com/article/view/10.21037/aes-22-35/rc).
Methods
A literature review was performed using PubMed to search all original research and review articles published through to June 2022. Some of the following search terms used included: “limbal stem cell deficiency”, “limbal stem cell deficiency diagnosis”, “ocular chemical injury”, “aniridia associated keratopathy”. Additional search terms are shown in Table 1.
Table 1
“Limbus Corneae” [Mesh] |
“Limbal stem cell deficiency” |
“Limbal stem cell deficiency” AND “anterior segment OCT” |
“Ocular chemical injury” |
“Limbal stem cell deficiency” AND “Glaucoma” |
“Aniridia associated keratopathy” |
“Limbal stem cell deficiency” AND “Stevens-Johnson Syndrome” |
“Limbal stem cell deficiency” AND “contact lens overuse” |
“Limbal stem cell deficiency” AND diagnosis |
OCT, optical coherence tomography.
Additional papers were identified by reviewing reference lists of relevant publications. All publications reviewed were in English and publications with relative low credibility were excluded. Data were extracted based on their relevance to the topic instead of implementing a systematic approach to paper selection. More details of the method are shown in Table 2.
Table 2
Items | Specification |
---|---|
Date of search | 3/1/2022–6/18/2022 |
Databases and other sources searched | PubMed |
Search terms used | See Table 1 |
Timeframe | 1921–2022 |
Inclusion and exclusion criteria | Original research articles and reviews written in English were included. Articles that focused on management or surgical treatment of limbal stem cell deficiency were excluded |
Selection process | Jennifer C. W. Hu conducted the search and selection. Both authors met and agreed regarding the final literature choice |
Any additional considerations, if applicable | Some papers were identified by reviewing reference lists of relevant publications |
Causes of LSCD (5)
There are many causes of LSCD with the most common listed in Table 3. Epidemiologic studies have been conducted globally seeking to identify the most common causes of LSCD. For instance, at a single tertiary center, in a series of 738 eyes over 14 years, the most common causes of LSCD identified were aniridia (30.9%), chemical or thermal injuries (20.6%), contact lens (16.8%), and Stevens-Johnson syndrome (10.4%) (12). Of note, among unilateral cases, the most common cause of LSCD is chemical burns (13,14). Other causes include prior surgery (most often pterygium or glaucoma surgeries), drug toxicity, mucous membrane pemphigoid (MMP), and others as listed in Table 3 (13,15). Of note, all ophthalmologists should understand LSCD, given that many types of ophthalmic surgery can affect limbal stem cells (16). In later sections of this review, we discuss the epidemiology of some of the most common causes of LSCD, which often depends on the age of the patient, their occupation, and their other ocular co-morbidities.
Table 3
Traumatic |
Chemical injuries |
Contact lens use |
Limbus-involving surgery |
Drug induced [in conjunction with glaucoma surgery with mitomycin C and 5-fluorouracil (7), dupilumab (8)] |
External beam radiation (9,10) |
Infectious |
Microbial keratitis |
Allergic |
Vernal keratoconjunctivitis (11) |
Immune-mediated |
SJS |
GVHD |
MMP |
Genetic |
Aniridia |
Congenital epidermal dysplasia |
Keratitis associated with multiple endocrine deficiencies |
Xeroderma pigmentosa |
SJS, Stevens-Johnson syndrome; GVHD, graft vs. host disease; MMP, mucous membrane pemphigoid.
Figure 2 shows a case of severe LSCD in the setting of prior surgery, courtesy of Danielle Trief. In conditions of longstanding ophthalmic disease, there may be limbal stem cell loss due to changes in the microenvironment of the limbal stem cells through a combination of inflammatory cytokines and matrix metalloproteinases. For example, patients with bullous keratopathy (BK) can have LSCD, leading to impaired epithelialization. One study that looked at patients with BK who underwent a penetrating keratoplasty found that patients without corneal neovascularization (and therefore less likely to have LSCD) re-epithelialized after ~6 days compared to ~29 days in those who had corneal neovascularization noted pre-operatively (17). LSCD following infectious keratitis is not uncommon either, therefore patients should be counseled on the risk of graft failure following therapeutic penetrating keratoplasty in this setting as well (16).
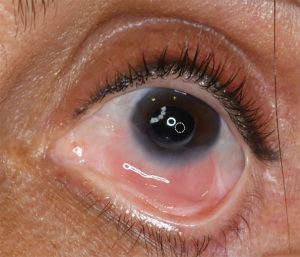
Diagnosis of LSCD
The diagnosis of LSCD is through a combination of clinical examination and imaging techniques, without any one method being clearly preferred. In a recent literature review of 46 studies of LSCD, clinical features (as described below) were used to diagnose LSCD in all studies and of those, 18 used one or more additional tests: impression cytology (17 studies), immunohistochemistry for epithelial markers (4 studies), and/or in vivo confocal microscopy (2 studies) (18). A comparison of different imaging modalities used for the diagnosis of LSCD is shown in Table 4.
Table 4
Diagnostic method | Advantages | Disadvantages |
---|---|---|
Impression cytology | Allows for identification of goblet cells, additional immunohistochemical staining | Subject to preparation quality |
Not available at all institutions | ||
Only evaluates 1–3 epithelial cell layers | ||
In vivo confocal microscopy | Ability to quantify cells and resolution of about 1–10 μm | Requires patient participation for 5–15 minutes including sitting with ocular lens in front of scanning laser |
Allows for imaging of all layers of the cornea, including deeper epithelial cell layers | Difficult in those with nystagmus | |
asOCT | No contact with the eye is needed | Lower resolution than confocal microscopy, 5 μm |
Scanning time of seconds |
asOCT, anterior segment optical coherence tomography.
Clinical examination
On slit lamp examination, as Vogt initially described, one may sometimes be able to appreciate the direct loss of the palisades. When staining the cornea with fluorescein, there may only be epithelial thinning in a vortex pattern or whorl-like epitheliopathy in mild disease (Figure 3A). In moderate disease, superficial neovascularization of the cornea and peripheral pannus becomes apparent (Figure 3B). In severe cases, this can lead to stromal neovascularization and scarring along with corneal opacification (Figure 4). In a global consensus (5) published from the Limbal Stem Cell Working Group by the Cornea Society from 2019, a clinical staging system was devised as shown in Table 5.
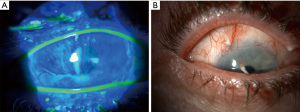
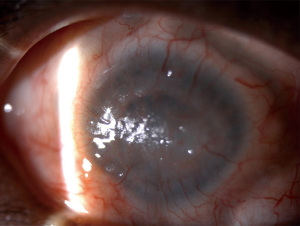
Table 5
Stage | Clinical findings | A | B | C |
---|---|---|---|---|
Stage I | Normal corneal epithelium within the central 5 mm zone of the cornea | <50% of limbal involvement | ≥50% but <100% limbal involvement | 100% of limbal involvement |
Stage II | The central 5 mm zone of the cornea is affected | <50% of limbal involvement | ≥50% but <100% limbal involvement | |
Stage III | The entire corneal surface is affected |
LSCD, limbal stem cell deficiency.
A separate clinical grading scale proposed by Aravena et al. (15) further correlated clinical signs with confocal microscopy imaging (thereby quantifying the basal epithelial cells). They proposed a score of 1 to 4 depending on the clock hour of limbal involvement, the extent of corneal surface involved, and whether there was encroachment within 5mm of the central visual axis. A total score was composed as the total of these 3 factors with the stages of LSCD classified as mild (2 to 4 points), moderate (5 to 7 points), and severe (8 to 10 points) based on the clinical grading. In control eyes, basal epithelial cell density (measured by cells/mm2) was 9,252 while compared to those that were graded clinically as having mild, moderate, and severe LSCD in which the cell density was 7,121, 5,512, and 2,821, respectively. This represented a clinically significant difference from controls (15). The use of imaging techniques such as confocal may augment our ability to differentiate different severities of LSCD.
Confocal imaging
Confocal imaging allows for the in vivo imaging of various different cell populations with a lateral resolution of 1–2 µm and an axial resolution of 5–10 µm (19). Patients must be able to sit for several minutes and tolerate a lens to be placed on the surface of the eye to image the various layers of the cornea. Compared to its use in the diagnosis and management of Fuchs’ or other endothelial cell pathologies, it is less readily used in the diagnosis of LSCD. Normal corneal epithelium is characterized by multiple layers of cells with large polygonal superficial flat cells while the basal layer of cells contains more hyporeflective cytoplasm and bright borders (20). Corneal stem cells are located at the basal layer of the limbus. Conjunctival epithelium has a more cuboid morphology with a more hyper-reflective cytoplasm (19). While corneal basal epithelial cells may be differentiated from conjunctival epithelial cells, there is no set number for a normal basal epithelial cell density with estimates ranging from 6,400 to 8,000 cells/mm2. It has been shown that confocal imaging can also be directed at the limbus itself, showing the complete loss of the POV in severe cases (20). However, because this is also a normal process of aging, pure definitions based only on cell counts or presence or absence of POV are inaccurate and must be correlated with clinical history.
Impression cytology
Impression cytology is considered by some to be the gold standard in diagnosing LSCD, although it is not readily used in clinical practice. In this method, a cellulose acetate filter paper, nitrocellulose membrane or Biopore membrane is applied to the ocular surface to remove 1–3 layers of ocular surface epithelium (21). The specimens can then be subjected to various stains including hematoxylin and eosin (H&E), Periodic acid Schiff, Papanicolaou, or Giemsa staining to evaluate for goblet and epithelial cells. The presence of conjunctival goblet cells indicates conjunctivalization, correlating to one of the clinical hallmarks of LSCD. Beyond traditional histologic stains, more specific markers are being investigated in order to more definitively define cells as corneal or conjunctival. Cytokeratin 3 is thought to be specific to corneal epithelium while cytokeratin 19 is thought to be specific to conjunctival epithelium (22). One of the limitations of this method, however, is that good quality cytology is variable—in one study, only 42% of sampled eyes could be graded due to limitations in having “good quality” cytology (22).
Anterior segment optical coherence tomography (asOCT)
asOCT is being investigated as a novel method of evaluating and diagnosing LSCD. This imaging modality offers the advantage of requiring no contact with the patient’s eye and rapid evaluation compared to the aforementioned methods. In evaluating the central cornea in patients with LSCD, it was found that there was a significant reduction in epithelial thickness and maximal limbal epithelial thickness in patients with LSCD compared to controls which may provide a useful clinical measure in patients (23). Additionally, asOCT can be used to directly image the POV. In patients with LSCD, it was found that there was loss of the smooth interface between the conjunctival epithelium and stroma, dilated conjunctival stromal vessels, and a decreased POV density (24,25).
Chemical injury
Epidemiology
In the United States (US), the American Academy of Ophthalmology Intelligent Research in Sight (IRIS) Registry is a clinical data registry of over 59 million unique patients. Through a retrospective study of the registry, the incidence of ocular burns was estimated to be 65 new cases per 100,000 new diagnoses in 2013, rising slightly to 78 cases per 100,000 in 2017 (26). The mean age of the 64,760 unique patients who had suffered chemical injuries was 48 years old, though a bimodal distribution was noted with many patients under the age of 1 years old. Alkali injuries tended to be more common overall compared to acidic injuries (27,28). Figure 5 shows a patient with an alkali injury due to plaster lime in the workplace. The most common locations of chemical injuries are in the workplace (29) and within workplace injuries, they are the 2nd most common injury (after metallic foreign bodies) (30). In addition, these injuries were more common among individuals in the lowest and second-lowest income quartiles (≤$48,749) (28). In the Nationwide Emergency Department Sample, emergency department (ED) charges in the US over a 3-year period due to chemical burn injuries totaled $106.7 million (28).
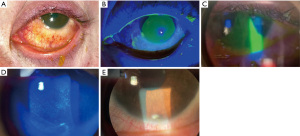
In comparison with unintentional chemical injuries, chemical injuries can also be due to assault or warfare and these injuries tend to have more severe outcomes. In a prospective study of 11,683 patients who presented to an eye infirmary ED, of the 98 acute chemical injuries, there were only 4 cases which reached the highest level of severity, Roper-Hall grade IV (Dua grade IV–VI). All four of these cases were cases of assaults with ammonia and progressed to complete LSCD (27). Similarly, in another series of the severe ocular chemical injuries (either grade III or IV on the Roper-Hall grading system), 16/25 cases were assault (31). Given both the severe visual outcome along with the emotional toll, this has led to countries across the world to creating new legislation to address formal legal definitions of chemical assault, controlling the sale of chemicals, facilitating justice, and support for survivors (32).
Clinical assessment
Acute clinical assessment of chemical injuries is important in determining ultimate prognosis. Initially, the Roper-Hall scale graded injuries on a scale from I to IV depending on the involvement of limbal ischemia and corneal opacity. Grade I was characterized by no limbal ischemia with only corneal epithelial damage and with a good prognosis while grade IV involved greater than 1/2 limbal ischemia with an opaque cornea and conferred a poor prognosis. Grade II involved between <1/3 limbal ischemia and grade III between 1/3 and 1/2 limbal ischemia. While the Roper-Hall classification has been in use since 1965, it has been argued that with newer management strategies, such as earlier amniotic membrane transplantation (AMT) or limbal stem cell transplantation, that even eyes with up to 75% limbal ischemia (historically grade IV on the Roper-Hall classification) may still do well (6,33). Dua et al. (6) proposed a new grading scheme which subdivided group IV by Roper-Hall into 3 subclasses: those with 50–75% limbal involvement (Dua grade IV), 75–100% involvement (Dua grade V), and 100% involvement (Dua grade VI) and found that even in those eyes that were historically categorized as Roper-Hall group IV injuries compared to Dua group VI had significantly different final outcomes. While early AMT placement does appear to provide benefit for Dua grade IV and V injury, there was no difference in final outcome for eyes with VI injury, or 100% clock-hour involvement, furthering our understanding that while recovery is possible (even likely) as long as there is 75% limbal involvement, 100% involvement can have devastating outcomes.
Management
Luckily, most cases of chemical injury will have a return to baseline visual acuity before 1 year, though there may be significant changes in the immediate period following injury (26). Identification of the agent is often not possible (28), but attempts should be made to identify the agent itself, the length of contact with the eye, and the strength of the solution. The pH of the eye should be checked and periodically evaluated while flushing the eye. The eyelids should be everted to identify any material which may continue to seed the eye. Greater risk for poorer best corrected visual acuity (BCVA) include older age, worse initial BCVA, and irrigation greater than 24 hours after injury (29).
Additional treatment will vary depending on the severity of the injury. A topical antibiotic ointment or drop, such as a fourth generation fluoroquinolone is helpful to prevent infection. Topical steroids such as prednisolone should be used initially to treat inflammation. In cases where significant limbal ischemia is noted, the use of doxycycline and vitamin C to prevent corneal melt are generally accepted. Should the damage progress into the anterior chamber, patients may have a rise in intraocular pressure (IOP). Attempts should be made to lower the IOP orally to prevent additional drop toxicity with a healing epithelium. Furthermore, cycloplegia may help with comfort. In patients with severe chemical injuries, amniotic membrane placement should be considered (34).
Some argue that there is a role for limbal stem cell transplantation in severe chemical injury either in the acute term (immediately following vascularization of the nearby conjunctiva to support a graft) or in the chronic phase. In one case series of 6 patients, both acute and chronic LSCD patients had improvement in their visual acuity and comfort after limbal stem cell transplantation from the contralateral eye (2–3 clock hours in size) (33).
Stevens-Johnson syndrome (SJS) and toxic epidermal necrolysis (TEN)
SJS is a severe, life-threatening disease characterized by sloughing of epithelial necrosis, oftentimes triggered by exposure to a new drug. When there is <10% body surface involvement, this is characterized as SJS while TEN is due to >30% involvement. Between 10% and 30% body surface involvement, there is overlap between SJS and TEN. The most common offending agents are antibiotics (sulfonamides most commonly) and anti-epileptics, followed by non-steroidal anti-inflammatory drugs (NSAIDs) and allopurinol (35). Other causes may include Mycoplasma pneumoniae infection, though these cases generally have less severe clinical manifestations (36). These patients may be critically ill, requiring hospitalization in burn units. For ophthalmologists, it is the standard of care to examine patients within 24–48 hours as there can be long-term morbidity if not properly monitored, with rates of ocular involvement ranging between 53–88% of cases in adults (36-38). The incidence of ocular involvement of pediatric patients with SJS/TEN was 32% (39).
Epidemiology
In a survey of 961 hospital-based US EDs between July 1, 2010, and June 30, 2012, a mean of 3,834 new SJS-spectrum cases per year were identified, correlating to an incidence rate of 12.35 new cases per million per year. In the United Kingdom, in incidence rate of 5.76 cases per million per year was identified (40). In both the US and Canada, there is now approval for the use of some human leukocyte antigen (HLA) genetic variant identification to aid in identifying patients at higher risk for SJS when exposed to particular medications. For instance, it is recommended that patients being considered for allopurinol should be tested for HLA-B*58:01 and carbamazepine HLA-B*15:02 (41) in an effort to reduce the rates of SJS.
Pathophysiology
The exact pathophysiology of SJS is not completely understood. Biopsies of the skin lesions are characterized by infiltration of the dermal tissues with lymphocytes and keratinocyte necrosis (36). Originally characterized as a type IV hypersensitivity reaction, additional mechanisms leading to keratinocyte necrosis have also been proposed including activation of Fas ligands (a surface molecule responsible for apoptosis) or perforin activation by natural killer cells (42). It has been found that in the eye, early on there may be epithelial sloughing and hemidesmosome loss even without the presence of immune cells. Early corneal keratinocytes have basal vacuolization despite having no immune cells present, suggesting that there may be cytokine dysregulation in the early phases of the disease prior to immune infiltration (43). In later phases of the disease, CD8+ cytotoxic lymphocytes infiltrate and target keratinocytes (43). It has been suggested that in the chronic phase of the disease, there are continued neutrophil presence in conjunctival tissue which may drive the continued immune dysregulation and lead to limbal stem cell compromise (44).
Clinical assessment and management
Patients can be categorized into 4 different grades (ranging from 0 to 3). In patients with grade 0, there is no ocular involvement and prophylactic artificial tears are recommended. In grade 1, there is conjunctival hyperemia but no corneal involvement, and topical antibiotic 3×/day, topical steroid 6×/day, topical steroid ointment 6×/day, and artificial tears every hour are recommended. In patients with grade 2, where there is corneal involvement but without any membranes, Prokera or AMT should be pursued (in addition to the grade 1 treatments). In grade 3, the same topical therapy as before should be used along with AMT. Long-term complication were significantly less likely when adhering to the treatments above compared to without strict grading criteria (35). Others have found early AMT intervention can provide excellent visual outcomes—in a study of 9/10 extremely severe cases, the final BCVA was 20/20 with 3 of 10 having moderate scarring of the tarsal conjunctiva and lid margins (45). Infectious keratitis may also occur in the setting of SJS, with one study identifying it in 35% of patients (18 eyes, 14 patients), highlighting the need for additional antibiotic prophylaxis (46).
Aniridia
Epidemiology
Aniridia is caused by a mutation in PAX6, leading to a host of different eye abnormalities in addition to the classic absence of the iris. It is inherited in an autosomal dominant fashion and usually the result of a nonsense mutation in one copy of the gene, with many causative mutations having been identified (47). The estimated incidence is 1 in 40,000 live births (48). Because of the nearby WT1 locus, patients often have congruous deletions and therefore are at risk of Wilms tumor (one of the four major components of WAGR syndrome, an acronym for Wilms tumor, aniridia, genitourinary problems, and developmental delay) (49). Patients with aniridia often have additional ocular consequences such as macular and optic nerve hypoplasia and glaucoma, which may all contribute to visual disability. Rates of aniridia associated keratopathy (AAK) range between 20% to over 80% (50,51). Presentation is often symmetric, though not always (52).
Pathophysiology
Patients with aniridia have progressive corneal opacification throughout life, thought to be due to LSCD. In multiple studies of patients with confirmed PAX6 mutations, there have been significantly decreased basal epithelial cell counts (by in vivo confocal microscopy) along with loss of the palisades correlating with worsened keratopathy (53,54). However, some have proposed that due to the widespread expression of PAX6, the keratopathy associated with aniridia may be due to a combination of not only LSCD, but also corneal stroma changes, mechanical changes in the limbal stem cell niche, and altered inflammatory of gene profiles of the adjacent conjunctival epithelial cells (47,51,55).
Clinical assessment and management
The clinical severity of AAK may be variable. In a cross-sectional study of 92 eyes of 47 patients with aniridia (average age 48), 12 eyes (13%) were classified as stage I AAK, 33 eyes (35.9%) were stage II, 25 eyes (27.2%) were stage III, 17 eyes (18.5%) were stage IV, and 5 eyes (5.4%) were stage V (56). Stage I was defined as only abnormal peripheral epithelium as assessed by fluorescein. Stage II was characterized by centripetalization of the epithelial changes however not yet center involving. Stage III meant that central corneal epithelial changes were present with some peripheral neovascularization superficially. Stage IV was defined as neovascularization superficially over the entire cornea and stage V was defined as abnormal corneal epithelium throughout with deep stromal scarring.
Identification of missense and non-coding mutants may help prognosticate patient’s clinical course, which has become a greater reality in clinical practice as the cost of genetic testing decreases. For instance, in one study AAK was found to be an age-dependent process in patients with premature termination codons or C-terminal extension mutations while other mutation types were age-independent or produced a non-progressive keratopathy (57). These results may provide clinicians with greater ability to prognosticate and provide anticipatory guidance in addition to identifying the type and timing of other treatments of LSCD.
Given the increasingly prevalent use of genetic testing, patients are being identified with causative mutations despite having minimal iris abnormalities and these patients may benefit from earlier intervention. Treatments in mild cases may include serum drops and AMT to improve surface quality, though patients often suffer recurrence of the keratopathy (58). Even with limbal stem cell transplantation or penetrating keratoplasty, the keratopathy often returns, highlighting the ongoing dysregulation (59). Keratoprostheses (KPro) may be an option and can be considered as first line; in a study of 26 eyes (of which 76% of cases the KPro was the primary corneal procedure), the KPro retention rate was 77%. However, there was a high rate of progression to glaucoma (88%), therefore transplanted patients must be monitored carefully (60).
Contact lens wear
Epidemiology
Contact lens wearers have a range of mild to severe LSCD. There is an estimated 125 million contact lens wearers in the US, therefore this represents a significant population of patients with LSCD (61). It is most commonly seen with soft contact lens use, with an estimated prevalence among contact lens wearers of around 2–5% (61). The duration of contact lens use before development of LSCD ranges between 14.1–17 years (62-64). Figure 6 shows one patient with severe LSCD in the setting of contact lens use.
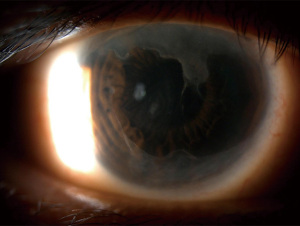
Pathophysiology
It is unclear whether the mechanical irritation/friction of the contact lens causes limbal stem cell injury or whether there is a change in the micro-environment due to preservatives or additives which the eye is exposed to through the lens solutions (65). Another theory posited is that the low-oxygen environment created by the contact lenses is unfavorable for the survival limbal stem cells (66).
Treatment
The first and primary treatment is stopping the usage of soft contact lenses. In many patients, stopping contact lens use alone may resolve epitheliopathy. Jeng et al. reported that in 11/18 eyes cessation of the contact lens led to resolution of the epitheliopathy and 5 required the addition of topical corticosteroid (64). Other treatment options include cyclosporine (67) or autologous serum tears (68).
Glaucoma
Epidemiology
Patients with primary open angle glaucoma (POAG) on topical therapy and/or history of glaucoma surgery are both at increased risk for LSCD. Given that the prevalence in the US alone is estimated to be 2.1% (affecting approximately 2.9 million individuals), this represents a large population at risk (69). Other primary eye conditions such as aniridia also predispose patients to glaucoma, further compounding the risk of LSCD. In a study of 434 eyes with LSCD, 35% of patients had glaucoma or ocular hypertension (OHT) (12).
Pathophysiology
There are multiple possible contributions to LSCD in patients with glaucoma. In patients who undergo surgery, there is a strong correlation between the location of corneal neovascularization and location of the glaucoma surgery (both trabeculectomy and aqueous shunt) (7). These procedures are thought to induce direct mechanical trauma to the cells, with more than one surgery being correlated with more severe LSCD (7). The combined use with anti-metabolites during this surgery to promote bleb formation and decrease wound healing has also been proposed to affect the microenvironment of the limbal stem cells (7,70). Furthermore, the topical drop therapies which patients are on chronically can be correlated with LSCD severity as well. In a study using in vivo confocal microscopy, patients with glaucoma medications (either one or two drops) were found to have higher dendritic cell density suggesting inflammation-mediated destruction of limbal stem cells, though interestingly the POV appeared similar between the glaucoma patients and controls (71). The infiltration of HLA-DR+ and IL-6+ cells as seen with impression cytology also suggests an increase in general inflammation which may contribute to a gradual loss of limbal stem cells over time (71). In a prospective, randomized two-year trial, treatment naïve glaucoma patients were put on preservative-free (PF), polyquad (PQ) and benzalkonium chloride (BAK) drop therapy and those with BAK preserved drops were found to have increased immune mediators compared as measured by impression cytology, mRNA, and quantitative polymerase chain reaction (qPCR) suggesting that it is the preservative which drives immune dysregulation (72).
Management
Given the progressive, blinding nature of glaucoma, surgical intervention may be the only option to prevent vision loss therefore it is important to be aware that patients may be at risk of developing LSCD. It is currently unclear the exact role and best practice in using anti-metabolites in preventing LSCD. It may be helpful in such patients to try to use preservative free formulations when possible in patients with glaucoma, to minimize their risk of LSCD (73).
Discussion
Future perspectives
In the last century, from the first description of the POV to the sophistication of in vivo confocal microscopy and asOCT, our ability to diagnosis, assess, and manage LSCD has grown tremendously. While clinical staging definitions were most recently reviewed by the Cornea Society in 2019, there remains opportunity to standardize and make use of the growing imaging modalities available to ophthalmologists for both diagnosis and monitoring of treatments for LSCD. For the corneal specialist, it is not only imperative to be able to recognize and treat the conditions which cause LSCD. Conditions such as aniridia highlight the ways in which advances in genetic sequencing may provide additional prognostication and aid in managing these patients in pediatric and adult populations. Chemical injuries represent a large financial burden and an area of opportunity to educate even non-ophthalmologic healthcare providers management to prevent severe LSCD. Taken together, these conditions cross a diverse set of patient populations and ophthalmic co-morbidities and should be of interest to all healthcare providers and ophthalmologists.
Limitations
This narrative review is meant to provide a comprehensive and critical synthesis of information regarding LSCD diagnosis and non-surgical management. It is limited by its use of one database and inclusion of articles which were most relevant and credible at the discretion of the authors. However, it is meant to provide a framework for understanding this complex and prevalent disease which is of interest for both corneal specialists and the general ophthalmologist.
Conclusions
LSCD has experienced a significant growth in its understanding and treatment. From the earliest studies looking at the palisades of Vogt to the newest surgical techniques, LSCD represents an oftentimes treatable condition which all ocular healthcare professions should be aware of. Its diagnosis is mainly clinical, aided by additional tools such as confocal microscopy, impression cytology, and asOCT. The most common causes include chemical injury, SJS/TEN, aniridia, contact lens overuse, glaucoma which each have unique demographic factors and management strategies.
Acknowledgments
Funding: None.
Footnote
Provenance and Peer Review: This article was commissioned by the Guest Editors (Joann Kang and Roy S. Chuck) for the series “Ocular Surface Reconstruction/Transplantation” published in Annals of Eye Science. The article has undergone external peer review.
Reporting Checklist: The authors have completed the Narrative Review reporting checklist. Available at https://aes.amegroups.com/article/view/10.21037/aes-22-35/rc
Peer Review File: Available at https://aes.amegroups.com/article/view/10.21037/aes-22-35/prf
Conflicts of Interest: Both authors have completed the ICMJE uniform disclosure form (available at https://aes.amegroups.com/article/view/10.21037/aes-22-35/coif). The series “Ocular Surface Reconstruction/Transplantation” was commissioned by the editorial office without any funding or sponsorship. The authors have no other conflicts of interest to declare.
Ethical Statement: The authors are accountable for all aspects of the work in ensuring that questions related to the accuracy or integrity of any part of the work are appropriately investigated and resolved.
Open Access Statement: This is an Open Access article distributed in accordance with the Creative Commons Attribution-NonCommercial-NoDerivs 4.0 International License (CC BY-NC-ND 4.0), which permits the non-commercial replication and distribution of the article with the strict proviso that no changes or edits are made and the original work is properly cited (including links to both the formal publication through the relevant DOI and the license). See: https://creativecommons.org/licenses/by-nc-nd/4.0/.
References
- Vogt A. Atlas of the Slitlamp-Microscopy of the Living Eye [Internet]. 1st ed. Springer-Verlag Berlin Heidelberg; [cited 2022 May 17]; 1921:154. Available online: https://link-springer-com.ezproxy.cul.columbia.edu/book/10.1007/978-3-662-26677-9
- Cotsarelis G, Cheng SZ, Dong G, et al. Existence of slow-cycling limbal epithelial basal cells that can be preferentially stimulated to proliferate: implications on epithelial stem cells. Cell 1989;57:201-9. [Crossref] [PubMed]
- Auran JD, Koester CJ, Kleiman NJ, et al. Scanning slit confocal microscopic observation of cell morphology and movement within the normal human anterior cornea. Ophthalmology 1995;102:33-41. [Crossref] [PubMed]
- Nagasaki T, Zhao J. Centripetal movement of corneal epithelial cells in the normal adult mouse. Invest Ophthalmol Vis Sci 2003;44:558-66. [Crossref] [PubMed]
- Deng SX, Borderie V, Chan CC, et al. Global Consensus on Definition, Classification, Diagnosis, and Staging of Limbal Stem Cell Deficiency. Cornea 2019;38:364-75. [Crossref] [PubMed]
- Dua HS, King AJ, Joseph A. A new classification of ocular surface burns. Br J Ophthalmol 2001;85:1379-83. [Crossref] [PubMed]
- Sun Y, Yung M, Huang L, et al. Limbal Stem Cell Deficiency After Glaucoma Surgery. Cornea 2020;39:566-72. [Crossref] [PubMed]
- Mehta U, Farid M. Dupilumab Induced Limbal Stem Cell Deficiency. Int Med Case Rep J 2021;14:275-8. [Crossref] [PubMed]
- Scholz SL, Hérault J, Stang A, et al. Proton radiotherapy in advanced malignant melanoma of the conjunctiva. Graefes Arch Clin Exp Ophthalmol 2019;257:1309-18. [Crossref] [PubMed]
- Ting DSJ, Rana-Rahman R, Ng JY, et al. Clinical Spectrum and Outcomes of Ocular and Periocular Complications following External-Beam Radiotherapy for Inoperable Malignant Maxillary Sinus Tumors. Ocul Oncol Pathol 2021;7:36-43. [Crossref] [PubMed]
- Sangwan VS, Jain V, Vemuganti GK, et al. Vernal keratoconjunctivitis with limbal stem cell deficiency. Cornea 2011;30:491-6. [Crossref] [PubMed]
- Cheung AY, Sarnicola E, Denny MR, et al. Limbal Stem Cell Deficiency: Demographics and Clinical Characteristics of a Large Retrospective Series at a Single Tertiary Referral Center. Cornea 2021;40:1525-31. [Crossref] [PubMed]
- Vazirani J, Nair D, Shanbhag S, et al. Limbal Stem Cell Deficiency-Demography and Underlying Causes. Am J Ophthalmol 2018;188:99-103. [Crossref] [PubMed]
- Cartes C, Lako M, Figueiredo FC. Referral Patterns of Patients with Limbal Stem Cell Deficiency to a Specialized Tertiary Center in the United Kingdom. Ophthalmol Ther 2021;10:535-45. [Crossref] [PubMed]
- Aravena C, Bozkurt K, Chuephanich P, et al. Classification of Limbal Stem Cell Deficiency Using Clinical and Confocal Grading. Cornea 2019;38:1-7. [Crossref] [PubMed]
- Sridhar MS, Vemuganti GK, Bansal AK, et al. Impression cytology-proven corneal stem cell deficiency in patients after surgeries involving the limbus. Cornea 2001;20:145-8. [Crossref] [PubMed]
- Uchino Y, Goto E, Takano Y, et al. Long-standing bullous keratopathy is associated with peripheral conjunctivalization and limbal deficiency. Ophthalmology 2006;113:1098-101. [Crossref] [PubMed]
- Jawaheer L, Anijeet D, Ramaesh K. Diagnostic criteria for limbal stem cell deficiency-a systematic literature review. Surv Ophthalmol 2017;62:522-32. [Crossref] [PubMed]
- Jalbert I, Stapleton F, Papas E, et al. In vivo confocal microscopy of the human cornea. Br J Ophthalmol 2003;87:225-36. [Crossref] [PubMed]
- Nubile M, Lanzini M, Miri A, et al. In vivo confocal microscopy in diagnosis of limbal stem cell deficiency. Am J Ophthalmol 2013;155:220-32. [Crossref] [PubMed]
- Singh R, Joseph A, Umapathy T, et al. Impression cytology of the ocular surface. Br J Ophthalmol 2005;89:1655-9. [Crossref] [PubMed]
- Donisi PM, Rama P, Fasolo A, et al. Analysis of limbal stem cell deficiency by corneal impression cytology. Cornea 2003;22:533-8. [Crossref] [PubMed]
- Liang Q, Le Q, Cordova DW, et al. Corneal Epithelial Thickness Measured Using Anterior Segment Optical Coherence Tomography as a Diagnostic Parameter for Limbal Stem Cell Deficiency. Am J Ophthalmol 2020;216:132-9. [Crossref] [PubMed]
- Chen YY, Sun YC, Tsai CY, et al. Spectral-domain optical coherence tomography for evaluating palisades of Vogt in ocular surface disorders with limbal involvement. Sci Rep 2021;11:12502. [Crossref] [PubMed]
- Kim KH, Mian SI. Diagnosis of corneal limbal stem cell deficiency. Curr Opin Ophthalmol 2017;28:355-62. [Crossref] [PubMed]
- Anchouche S, Hall N, Bal S, et al. Chemical and thermal ocular burns in the United States: An IRIS registry analysis. Ocul Surf 2021;21:345-7. [Crossref] [PubMed]
- Ghosh S, Salvador-Culla B, Kotagiri A, et al. Acute Chemical Eye Injury and Limbal Stem Cell Deficiency-A Prospective Study in the United Kingdom. Cornea 2019;38:8-12. [Crossref] [PubMed]
- Haring RS, Sheffield ID, Channa R, et al. Epidemiologic Trends of Chemical Ocular Burns in the United States. JAMA Ophthalmol 2016;134:1119-24. [Crossref] [PubMed]
- Li T, Jiang B, Zhou X. Clinical characteristics of patients hospitalized for ocular chemical injuries in Shanghai from 2012 to 2017. Int Ophthalmol 2020;40:909-16. [Crossref] [PubMed]
- Quesada JM, Lloves JM, Delgado DV. Ocular chemical burns in the workplace: Epidemiological characteristics. Burns 2020;46:1212-8. [Crossref] [PubMed]
- Hoffman JJ, Casswell EJ, Shortt AJ. Assault-related severe ocular chemical injury at a London ophthalmic referral hospital: a 3-year retrospective observational study. BMJ Open 2020;10:e038109. [Crossref] [PubMed]
- Kazerooni Y, Mishra B, Gibran N, et al. A systematic review and comprehensive legislative framework to address chemical assault globally. Health Policy Plan 2020;35:1188-207. [Crossref] [PubMed]
- Morgan S, Murray A. Limbal autotransplantation in the acute and chronic phases of severe chemical injuries. Eye (Lond) 1996;10:349-54. [Crossref] [PubMed]
- Eslani M, Baradaran-Rafii A, Cheung AY, et al. Amniotic Membrane Transplantation in Acute Severe Ocular Chemical Injury: A Randomized Clinical Trial. Am J Ophthalmol 2019;199:209-15. [Crossref] [PubMed]
- Shanbhag SS, Rashad R, Chodosh J, et al. Long-Term Effect of a Treatment Protocol for Acute Ocular Involvement in Stevens-Johnson Syndrome/Toxic Epidermal Necrolysis. Am J Ophthalmol 2019;208:331-41. [Crossref] [PubMed]
- Wetter DA, Camilleri MJ. Clinical, etiologic, and histopathologic features of Stevens-Johnson syndrome during an 8-year period at Mayo Clinic. Mayo Clin Proc 2010;85:131-8. [Crossref] [PubMed]
- Morales ME, Purdue GF, Verity SM, et al. Ophthalmic Manifestations of Stevens-Johnson Syndrome and Toxic Epidermal Necrolysis and Relation to SCORTEN. Am J Ophthalmol 2010;150:505-510.e1. [Crossref] [PubMed]
- Chow LLW, Shih KC, Chan JCY, et al. Comparison of the acute ocular manifestations of Stevens-Johnson syndrome and toxic epidermal necrolysis in Chinese eyes: a 15-year retrospective study. BMC Ophthalmol 2017;17:65. [Crossref] [PubMed]
- Choi SH, Kim MK, Oh JY. Corneal Limbal Stem Cell Deficiency in Children with Stevens-Johnson Syndrome. Am J Ophthalmol 2019;199:1-8. [Crossref] [PubMed]
- Frey N, Jossi J, Bodmer M, et al. The Epidemiology of Stevens-Johnson Syndrome and Toxic Epidermal Necrolysis in the UK. J Invest Dermatol 2017;137:1240-7. [Crossref] [PubMed]
- Chang WC, Abe R, Anderson P, et al. SJS/TEN 2019: From science to translation. J Dermatol Sci 2020;98:2-12. [Crossref] [PubMed]
- Hazin R, Ibrahimi OA, Hazin MI, et al. Stevens-Johnson syndrome: pathogenesis, diagnosis, and management. Ann Med 2008;40:129-38. [Crossref] [PubMed]
- Williams GP, Mudhar HS, Leyland M. Early pathological features of the cornea in toxic epidermal necrolysis. Br J Ophthalmol 2007;91:1129-32. [Crossref] [PubMed]
- Williams GP, Tomlins PJ, Denniston AK, et al. Elevation of conjunctival epithelial CD45INTCD11b+CD16+CD14− neutrophils in ocular Stevens-Johnson syndrome and toxic epidermal necrolysis. Invest Ophthalmol Vis Sci 2013;54:4578-85. [Crossref] [PubMed]
- Gregory DG. New Grading System and Treatment Guidelines for the Acute Ocular Manifestations of Stevens-Johnson Syndrome. Ophthalmology 2016;123:1653-8. [Crossref] [PubMed]
- Kang BS, Kim MK, Wee WR, et al. Infectious Keratitis in Limbal Stem Cell Deficiency: Stevens-Johnson Syndrome Versus Chemical Burn. Cornea 2016;35:51-5. [Crossref] [PubMed]
- Latta L, Figueiredo FC, Ashery-Padan R, et al. Pathophysiology of aniridia-associated keratopathy: Developmental aspects and unanswered questions. Ocul Surf 2021;22:245-66. [Crossref] [PubMed]
- Grønskov K, Olsen JH, Sand A, et al. Population-based risk estimates of Wilms tumor in sporadic aniridia. A comprehensive mutation screening procedure of PAX6 identifies 80% of mutations in aniridia. Hum Genet 2001;109:11-18. [Crossref] [PubMed]
- Wawrocka A, Krawczynski MR. The genetics of aniridia - simple things become complicated. J Appl Genet 2018;59:151-9. [Crossref] [PubMed]
- Farah CJ, Fries FN, Latta L, et al. An attempt to optimize the outcome of penetrating keratoplasty in congenital aniridia-associated keratopathy (AAK). Int Ophthalmol 2021;41:4091-8. [Crossref] [PubMed]
- Schlötzer-Schrehardt U, Latta L, Gießl A, et al. Dysfunction of the limbal epithelial stem cell niche in aniridia-associated keratopathy. Ocul Surf 2021;21:160-73. [Crossref] [PubMed]
- Lagali N, Wowra B, Dobrowolski D, et al. Stage-related central corneal epithelial transformation in congenital aniridia-associated keratopathy. Ocul Surf 2018;16:163-72. [Crossref] [PubMed]
- Lagali N, Edén U, Utheim TP, et al. In vivo morphology of the limbal palisades of vogt correlates with progressive stem cell deficiency in aniridia-related keratopathy. Invest Ophthalmol Vis Sci 2013;54:5333-42. [Crossref] [PubMed]
- Le Q, Deng SX, Xu J. In vivo confocal microscopy of congenital aniridia-associated keratopathy. Eye (Lond) 2013;27:763-6. [Crossref] [PubMed]
- Puangsricharern V, Tseng SC. Cytologic evidence of corneal diseases with limbal stem cell deficiency. Ophthalmology 1995;102:1476-85. [Crossref] [PubMed]
- Yazdanpanah G, Bohm KJ, Hassan OM, et al. Management of Congenital Aniridia-Associated Keratopathy: Long-Term Outcomes from a Tertiary Referral Center. Am J Ophthalmol 2020;210:8-18. [Crossref] [PubMed]
- Lagali N, Wowra B, Fries FN, et al. PAX6 Mutational Status Determines Aniridia-Associated Keratopathy Phenotype. Ophthalmology 2020;127:273-5. [Crossref] [PubMed]
- Ihnatko R, Eden U, Fagerholm P, et al. Congenital Aniridia and the Ocular Surface. Ocul Surf 2016;14:196-206. [Crossref] [PubMed]
- Skeens HM, Brooks BP, Holland EJ. Congenital aniridia variant: minimally abnormal irides with severe limbal stem cell deficiency. Ophthalmology 2011;118:1260-4. [Crossref] [PubMed]
- Hassanaly SI, Talajic JC, Harissi-Dagher M. Outcomes following Boston type 1 keratoprosthesis implantation in aniridia patients at the University of Montreal. Am J Ophthalmol 2014;158:270-276.e1. [Crossref] [PubMed]
- Rossen J, Amram A, Milani B, et al. Contact Lens-induced Limbal Stem Cell Deficiency. Ocul Surf 2016;14:419-34. [Crossref] [PubMed]
- Chan CC, Holland EJ. Severe limbal stem cell deficiency from contact lens wear: patient clinical features. Am J Ophthalmol 2013;155:544-549.e2. [Crossref] [PubMed]
- Martin R. Corneal conjunctivalisation in long-standing contact lens wearers. Clin Exp Optom 2007;90:26-30. [Crossref] [PubMed]
- Jeng BH, Halfpenny CP, Meisler DM, et al. Management of focal limbal stem cell deficiency associated with soft contact lens wear. Cornea 2011;30:18-23. [Crossref] [PubMed]
- Stapleton F, Bakkar M, Carnt N, et al. CLEAR - Contact lens complications. Cont Lens Anterior Eye 2021;44:330-67. [Crossref] [PubMed]
- Takatori SC, Lazon de la Jara P, Holden B, et al. In vivo corneal oxygen uptake during soft-contact-lens wear. Invest Ophthalmol Vis Sci 2013;54:3472-9. [Crossref] [PubMed]
- Kim BY, Riaz KM, Bakhtiari P, et al. Medically reversible limbal stem cell disease: clinical features and management strategies. Ophthalmology 2014;121:2053-8. [Crossref] [PubMed]
- Yeh SI, Chu TW, Cheng HC, et al. The Use of Autologous Serum to Reverse Severe Contact Lens-induced Limbal Stem Cell Deficiency. Cornea 2020;39:736-41. [Crossref] [PubMed]
- Gupta P, Zhao D, Guallar E, et al. Prevalence of Glaucoma in the United States: The 2005-2008 National Health and Nutrition Examination Survey. Invest Ophthalmol Vis Sci 2016;57:2905-2913. [Crossref] [PubMed]
- Schwartz GS, Holland EJ. Iatrogenic limbal stem cell deficiency: when glaucoma management contributes to corneal disease. J Glaucoma 2001;10:443-5. [Crossref] [PubMed]
- Mastropasqua R, Agnifili L, Fasanella V, et al. Corneoscleral limbus in glaucoma patients: in vivo confocal microscopy and immunocytological study. Invest Ophthalmol Vis Sci 2015;56:2050-8. [Crossref] [PubMed]
- Mohammed I, Kulkarni B, Faraj LA, et al. Profiling ocular surface responses to preserved and non-preserved topical glaucoma medications: A 2-year randomized evaluation study. Clin Exp Ophthalmol 2020;48:973-82. [Crossref] [PubMed]
- Zhivov A, Kraak R, Bergter H, et al. Influence of benzalkonium chloride on langerhans cells in corneal epithelium and development of dry eye in healthy volunteers. Curr Eye Res 2010;35:762-9. [Crossref] [PubMed]
Cite this article as: Hu JCW, Trief D. A narrative review of limbal stem cell deficiency & severe ocular surface disease. Ann Eye Sci 2023;8:13.