Application of optical coherence tomography in hereditary, toxic and metabolic optic neuropathies
Introduction
Most metabolic, toxic and hereditary optic neuropathies are thought to arise from mitochondrial dysfunction. They present with similar symptoms, including painless central or cecocentral scotomas, reduced visual acuity and dyschromatopsia. Vision changes are typically bilateral, or unilateral with sequential involvement of the second eye. The onset can be sudden or insidious, depending on the etiology, and visual outcomes can range from complete recovery to no light perception vision. Exam characteristically shows selective thinning or pallor of the temporal aspect of the optic nerve commensurate with the extent of optic nerve injury, especially in the setting of prolonged disease.
These characteristics stem from preferential damage to the papillomacular bundle (PMB) in mitochondrial optic neuropathies. Retinal ganglion cell (RGC) axons course over the anterior surface of the retina in the retinal nerve fiber layer (RNFL) in distinct geographical bundles to converge on the optic nerve head. The PMB carries fibers from the macula to form the temporal aspect of the optic nerve. In contrast, the superior and inferior polar regions of the nerve are formed by fibers arising superior and inferior to the horizontal raphe temporal to the fovea, while the nasal optic nerve encompasses fibers from the nasal periphery (Figure 1). Therefore, damage to the PMB impacts macular function and results in temporal optic nerve pallor on fundus examination and RNFL thinning with OCT.
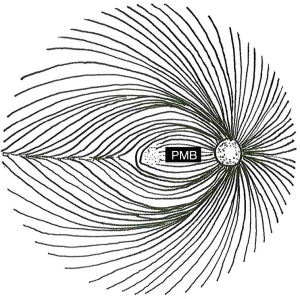
Several atypical features of the axons within the PMB place them under increased metabolic stress. They are unmyelinated and require large amounts of energy to propagate action potentials relative to the saltatory conduction present in myelinated nerves. The PMB also carries a relatively high proportion of axons arising from small-caliber, rapid-firing parvocellular RGCs (1-4). Small-caliber axons are thought to be more susceptible to metabolic stress, as models of mitochondrial optic neuropathy based on axon caliber accurately predict nerve fiber loss in pathologic sections from patients with mitochondrial optic neuropathy (2). This is attributed to the axon’s lower volume-to-surface-area ratio (which equates to having fewer mitochondria available to maintain membrane potentials). Finally, as the nerve fibers exit the eye, they make a sharp turn to pass through the lamina cribrosa. This configuration represents a “choke point” of restricted axoplasmic flow in which there are increased metabolic demands for intracellular transport. Histologic sections of the optic nerve demonstrate a high concentration of mitochondria in this area (5). Overall, the PMB appears to be more susceptible to damage in the setting of mitochondrial dysfunction due to the energetic demands of small-caliber, unmyelinated nerve fibers traveling in a physically constricted space. This helps explain why mitochondrial dysfunction (which should be present in every cell in the body) can selectively affect the optic nerve in general and the PMB fibers in particular.
Mitochondrial optic neuropathies can sometimes be difficult to distinguish from other causes of optic nerve injury including glaucoma, inflammatory disorders, ischemia, compression, trauma or malignancy. Often a thorough history and exam helps to narrow the differential diagnosis. Ancillary testing including visual fields, fundus autofluorescence and optical coherence tomography (OCT) can be helpful in equivocal cases, in addition to blood work and imaging. OCT is a non-invasive, relatively affordable imaging modality that allows for high-resolution, quantitative visualization of the optic nerve and macula. This technology allows for quantification of axonal and neuronal loss, which can serve as a valuable marker for progression of visual loss, as well as a predictor for visual recovery with treatment.
Several OCT modalities are helpful in the setting of mitochondrial optic neuropathies. Assessment of the RNFL with OCT provides objective measurement of sectoral swelling or atrophy of the optic nerve, which can be followed over time to track improvement or progression. Macular OCT with GC-IPL segmentation allows for quantification of the ganglion cell layer and inner plexiform layer (IPL). In the setting of acute injury, GC-IPL analysis is particularly useful, as GC-IPL thinning may be apparent while RNFL thinning is masked by active swelling or distention of the nerve fibers. In other conditions, including optic neuritis, GC-IPL thinning can precede measurable RNFL thinning even in the absence of swelling. This surrogate marker of neuronal integrity can also provide prognostic information and be followed over time to track progression. In patients with central scotoma, macular OCT also provides visualization of the outer retina, which is helpful in distinguishing between disease processes effecting the retina and optic nerve. A few early studies of OCT-angiography in hereditary optic neuropathy demonstrate detectable changes prior to the onset of RNFL thinning, suggesting that it may be a helpful technique as it becomes more widely available (6-8). Different hereditary, toxic and metabolic optic neuropathies manifest somewhat different findings on OCT, as discussed below.
Leber hereditary optic neuropathy (LHON) x
First described by Theodore Leber in 1871, LHON often causes sequential bilateral vision loss in young adults. The majority of cases (90–95%) are attributed to mitochondrial DNA mutations at position G11778A, T14484A, and G3460A, which effect NADH dehydrogenases (ND4, ND6, and ND1, respectively) (9,10). These dehydrogenases are complex I proteins that play a critical role in oxidative phosphorylation, neutralizing free radicals and regulating apoptosis (10). Prevalence varies somewhat between different populations, with the prevalence in Europe being roughly 1:30,000. LHON exhibits variable penetrance, with 50% of male mutation carriers and 10% of female carriers developing vision loss. The typical age of onset ranges from 15 to 35, but cases have been reported in all decades of life (9).
Clinically, LHON is characterized by the sequential, painless onset of dense cecocentral scotomas with dyschromatopsia. Visual acuity is typically reduced to 20/200 or less, and the fellow eye is almost always affected within the first year, most commonly within 6–8 weeks. Vision loss typically progresses for up to 6 months and then plateaus. Some visual improvement is possible, particularly in patients carrying the 14,484 mutation, for which a 37–71% chance of partial visual recovery has been reported, relative to 4% for the other mutations (9). Fundus exam at the time of onset typically shows thickening of the RNFL, hyperemia, peripapillary telangiectasias and mild tortuosity, although these findings are not always present. Fluorescein angiography characteristically shows the absence of leakage at the optic nerve head, which can help in distinguishing this process from other optic neuropathies.
Several longitudinal studies of patients with LHON have described characteristic RNFL changes on OCT. Asymptomatic carriers have statistically significant thickening of the temporal RNFL relative to age-matched controls, and a trend towards inferior RNFL thickening (11). This early thickening has been attributed to impairment of axoplasmic flow and likely represents early or baseline stress to the nerve fibers. At the time of symptom onset, there is typically predominant thickening of the superior and inferior RNFL, with normalization of the temporal RNFL as it begins to atrophy (12). These changes correspond to the appearance of disc edema, hyperemia and microvascular change on fundus exam. After 3 months, temporal thinning can be appreciated, and by 9 months superior and inferior thinning is apparent as well (13).
Progressive changes in the macula have been characterized in more recent case series of LHON patients. Macular OCT shows thinning of the inner macular ring and nasal peripheral macula in the first 3 months after symptom onset. This thinning then progresses to involve the temporal macula over the next 3 months, and by 12 months, diffuse macular thinning is evident, with average macular thickness of 251 µm compared with an average of 281 µm in controls (14). GC-IPL analysis appears to be even more sensitive to early changes in LHON. A small longitudinal case series of 4 patients demonstrated thinning of the GC-IPL in the nasal macula prior to symptom onset. In the first three months after the onset of symptoms, the GC-IPL thinning progressed to involve the inferior, then superior and temporal sectors with diffuse thinning of the average GC-IPL to 63 µm at 3 months. This thinning continued to progress to 57 µm at 6 months, then stabilized (7). See Figure 2 for a discussion of a case with typical progressive changes on OCT.
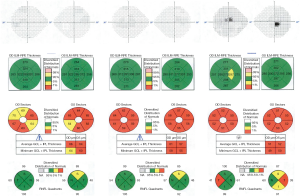
Recent case reports have indicated that changes in the radial peripapillary capillary (RPC) network can be appreciated in patients with LHON using OCT-angiography (15-17). This has been more thoroughly explored in a case series of 22 patients at different stages in disease progression. Capillary dropout in the RPC network was noted, with decreased vascular density occurring temporally in early disease stages, followed by inferior and superior capillary dropout, then diffuse dropout in chronic disease. The changes in vascularity corresponded with GC-IPL thinning, and preceded RNFL thinning (6). As OCT-angiography becomes more widely available, it may prove a useful correlate to GC-IPL in tracking disease progression and response to therapy, particularly in the early stages of the disease.
Given the sequential vision loss in LHON, there is a small window of opportunity in which prompt diagnosis may allow intervention prior to loss of vision in the fellow eye. A randomized, double-blinded, placebo-controlled trial demonstrated that idebenone 900 mg/d results in mild improvement of visual acuity outcomes in patients with discordant visual acuities at the onset of treatment, particularly in patients with the 11,778 and 3,460 mutations (18). Idebenone is not currently approved for treatment of LHON in the United States or Canada, but has been approved in the European Union for this purpose. Gene therapy is another emerging treatment option for LHON. Stage I/II clinical trials have been completed in 15 patients with the 11,778-mutation demonstrating a favorable safety profile, and stage III trials (RESCUE and REVERSE) are ongoing (19). Patients are likely to have maximal benefit from these treatment options with early diagnosis. Quantification of axonal and neuronal loss with OCT in general, and GC-IPL analysis in particular, can provide critical early diagnostic information for LHON, which will assist in identifying patients in the early stages of disease who may benefit the most from intervention as more treatments become available.
Autosomal dominant optic atrophy (ADOA)
ADOA, first described by Paul Kjer in 1957, typically presents earlier than LHON, with most patients developing symptoms in the first decade of life. Vision loss is bilateral, simultaneous and gradual in onset, and very slowly progressive over the course of decades. Patients most commonly exhibit reduced visual acuity, cecocentral scotomas and blue/yellow dyschromatopsia. The majority of patients retain visual acuity of 20/200 or better, with one third retaining 20/60 or better, and there is a high degree of variability in penetrance and expressivity within families (20). While cecocentral scotomas are most common, these patients can also present with bilateral superotemporal visual field loss that does not respect the vertical midline (21). The optic disc characteristically exhibits temporal pallor or excavation, which can progress to diffuse pallor later in the course of disease (22,23). Prevalence ranges from 1:10,000 to 1:50,000 worldwide, with the highest incidence in Denmark (24-26).
Mutations in the OPA1 gene are most frequently associated with ADOA, although cases attributed to OPA3 mutations have been reported as well (24). Over 100 different OPA1 mutations have been implicated in ADOA, and OPA1 mutations are identified in 32% to 89% of cases. Both missense and haplo-insufficiency mutations have been identified, with more severe atrophy associated with missense mutations (27). OPA1 encodes a dynamin-related GTPase that localizes to the mitochondrial inner membrane, and plays an important role in mitochondrial fusion, oxidative phosphorylation and apoptosis. Therefore, although ADOA follows a Mendelian inheritance pattern, it behaves like a mitochondrial optic neuropathy. Apoptosis of RGCs is a critical part of normal development, with an estimated reduction in RGC numbers from 2.2–2.5 to 1.5–1.7 million over the course of development in utero (28). Patients with vision loss and confirmed OPA1 mutations have optic discs that are smaller than those in age-matched controls, and more severe phenotypes are associated with smaller discs, suggesting that greater rates of apoptosis in RGCs may drive this disease (29,30). Histologic studies of patients of with ADOA are consistent with RGC dropout, demonstrating fibrosis and cell loss of the RGC layer, with associated myelin loss and increased fibrosis in the optic nerve and lateral geniculate nucleus (LGN) (31,32).
Analysis of RNFL thickness using OCT has consistently demonstrated a severe reduction in average RNFL thickness in patients with ADOA compared with healthy controls. This thinning affects the entire optic nerve, but like other mitochondrial-related optic neuropathies is most prominent temporally, with multiple cross-sectional studies demonstrating thinning that is most pronounced temporally and inferiorly, then superiorly, and least pronounced nasally (27,30,33-36). This characteristic pattern can help distinguish ADOA from other optic neuropathies presenting in childhood including glaucoma, inflammatory optic neuropathies and compressive lesions. RNFL thinning occurs with aging in the general population. Curiously, the rate of RNFL thinning from the first to eighth decade of life appeared comparable in patients with ADOA compared with healthy controls, as assessed in two cross-sectional studies (33,34). This suggests that pathologic RGC is established very early in life, perhaps even prenatally. Clinically, we have also observed that patients with ADOA tend to perform better on automated perimetry than patients with other optic neuropathies and comparable RNFL thinning, which could reflect improved cortical adaptation.
Noninvasive imaging of specific retinal layers in the setting of ADOA has been undertaken with macular OCT (37,38). This approach demonstrates thinning of the inner retinal layers (GCL, IPL), while the outer retinal layers (INL, OPL, ONL, ellipsoid zone, RPE) appear intact (27,37). In particular, GC-IPL thinning is seen early in the course of disease, and shows more pronounced changes than the RNFL (27,30). Therefore, macular OCT with GC-IPL analysis may helpful in the setting of childhood-onset vision loss, to distinguish between ADOA and retinal dystrophies. Correlations between visual acuity and OCT measures including RNFL thinning and GC-IPL thinning have been described (8,27,39). However, OCT is not used routinely for predicting the degree of subsequent vision loss.
Recent case-control studies using OCT-angiography in patients with ADOA have demonstrated reduced density of the temporal RPC network and macular superficial capillary plexus, which supply the RGCs. The deep capillary plexus and the foveal avascular zone were unaffected (8,40). These studies yielded conflicting results on the correlation between RPC density and visual acuity. There was a stronger correlation between visual acuity and GC-IPL thickness, suggesting that GC-IPL analysis may be the most useful OCT modality currently available for objectively tracking vision-affecting changes over time. It is unclear if the vascular changes noted are secondary to RGC loss, or if capillary dropout plays a role in the pathogenesis of ADOA. Future studies might explore OCT-angiography in younger patients or carriers to better define the progression of vascular changes early in the course of disease.
Other hereditary mitochondrial disorders
Optic neuropathy can occur in the context of other more rare mitochondrial syndromes, which have some overlapping genetic risk factors. Wolfram Syndrome, also known as DIDMOAD (diabetes insipidus, diabetes mellitus, optic atrophy, and deafness), has been attributed to mutations in WFS1 and WFS2, but there is also increased risk in carriers of mitochondrial mutations associated with LHON. Behr’s syndrome (optic atrophy, ataxia, mental retardation, urinary incontinence, pes cavus) is associated with OPA3 in some pedigrees. Optic atrophy has also been described as a feature of Friedrich’s ataxia, specific forms of spinocerebellar ataxia (SCA1 and SCA3), mitochondrial encephalopathy, lactic acidosis, and stroke-like episodes (MELAS), and chronic progressive external ophthalmoplegia (CPEO). A high level of suspicion for optic neuropathy should be maintained when patients diagnosed with these syndromes present with vision changes, particularly if the clinical presentation, exam and ancillary testing are consistent. In these cases, OCT can be a valuable adjunct to the examination, allowing more precise assessment of optic atrophy and progression over time, particularly in patients who cannot reliably perform psychophysical tests such as visual acuity and perimetry.
Ethambutol toxicity
Ethambutol is most commonly used in the treatment of Mycobacterium tuberculosis and Mycobacterium avium complex infections. Mitochondrial toxicity is thought to result from its activity as a metal chelator, as local depletion of copper at the mitochondria inhibits cytochrome C oxidase, slows oxidative phosphorylation and triggers apoptosis (41). Ethambutol-induced optic neuropathy occurs in a dose-responsive fashion. Under current recommended dosing regimens, 1% of patients develop toxicity, presenting with bilateral central vision loss, along with diminished color vision and contrast sensitivity (42,43). A subset of patients do not present with central or cecocentral scotomas, but instead develop junctional scotomas (Figure 3) or bitemporal hemianopsia that does not respect the vertical midline (44). The vision loss typically progresses for 1–2 months after discontinuing the medication, then gradually improves over the subsequent 6 months with partial or full recovery of vision.
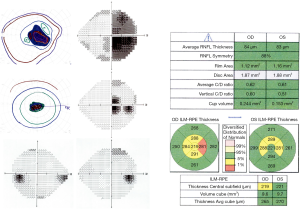
Several small case series have demonstrated that OCT of the RNFL is often normal near the time of symptom onset, with progressive thinning over the subsequent several months. This thinning is diffuse, with decreased average RNFL thickness (by 19–48%), but is most prominent temporally (with 19–72% thinning) (45-49). A small case series evaluating full thickness macular OCT demonstrated thinning throughout the inner macula, and outer nasal macula, which corresponds to the area of the PMB (50). The utility of GC-IPL thickness analysis as compared to RNFL thickness has also been assessed in multiple case series, which suggest that thinning of the GC-IPL appears earlier than RNFL thinning, and is a better predictor of visual outcome (51-53).
Early identification of toxicity is critical, as partial visual recovery is possible with discontinuation of the medication. Current screening recommendations for ethambutol-induced optic neuropathy include regular monitoring of visual acuity, color vision and visual fields (54). The role for OCT in screening asymptomatic patients is unclear. As detailed above, case reports have demonstrated that OCT detects minimal change in the RNFL at the time of symptom onset. Furthermore, prospective studies evaluating OCT of the RNFL have shown mixed results, with one study indicating an increase in average RNFL thickness, while two other studies reported sectoral thinning in a subset of patients (55-57). Assessment of GC-IPL thickness may be more promising as a screening tool, as GC-IPL changes have been documented prior to RNFL changes, and have been correlated with visual acuity as well.
Methanol toxicity
Methanol ingestion is associated with metabolic acidosis and optic neuropathy. The neuropathy may be secondary to demyelination of the optic nerve posterior to the lamina cribrosa (58). The methanol metabolite formic acid is a known inhibitor of mitochondrial cytochrome C oxidase, which is essential for oxidative phosphorylation and likely has a direct toxic effect on retinal nerve fibers as well (59). While accidental or intentional ingestion does occur in isolated cases, there have periodically been outbreaks of toxicity associated with commercial distribution of tainted ethanol, which has allowed for longitudinal studies of groups of patients with methanol intoxication.
Patients with methanol intoxication experience bilateral, acute onset, decreased visual acuity (which can be as severe as no light perception), visual field changes and photophobia, followed by gradual improvement over the subsequent weeks. In a retrospective study of 122 patients impacted by an outbreak of methanol poisoning in Ahmedabad, India in 2009, disc edema was noted at the time of presentation in 42% of patients, and optic nerve pallor in 20% of patients after 3 months (60). Visual prognosis depended on the visual acuity and serum pH at presentation, with a serum pH <7.3 associated with an increased risk of chronic RNFL thinning (OR 11.65) (60,61). Chronic changes were also noted in 42 patients involved in a mass methanol poisoning in the Czech Republic in 2012, with, 40% of patients developing chronic visual sequela. These patients also demonstrated RNFL thinning at 5 months, while only rare borderline changes were noted in patients who returned to baseline vision (61,62). Visual acuity remained relatively stable over the 4-year follow-up period, although there was some progression in RNFL thinning in severe cases. GC-IPL analysis has not been conducted in a large study group, but a case study of a single patient with persistent small cecocentral scotomas reported normal OCT RNFL, with mild thinning of the nasal macula GC-IPL at 1 month, which was more prominent at 8 months (63).
The presence or absence of RNFL and GC-IPL thinning on OCT may be helpful in counseling patients on visual prognosis, particularly several months after ingestion. As in other forms of mitochondrial optic neuropathy, changes in the GC-IPL may precede detectable RNFL changes. No intervention has been definitively shown to improve prognosis, although steroids and erythropoietin have both been reported anecdotally (64,65).
Amiodarone toxicity
Amiodarone is an anti-arrhythmic that is associated with optic neuropathy in 2% of patients. Amiodarone-associated optic neuropathy has been attributed to lamellar inclusion bodies within the optic nerve oligodendrocytes secondary to lysosomal dysfunction, rather than mitochondrial dysfunction (66). The average time to onset in a meta-analysis of 296 patients was 6 to 9 months after initiation of therapy (67). Classically, patients experience insidious, mild, bilateral vision loss (to 20/30 on average). Disc edema is documented in 85% of patients. However, the clinical presentation can vary. Some patients develop acute, or more severe loss of vision, and 35% present with unilateral symptoms and disc edema (68). These unilateral cases can be difficult to distinguish from NAION, particularly as patients on amiodarone are likely to have vasculopathic risk factors for NAION. The key distinguishing feature is that disc edema in NAION typically resolves after several weeks, while the edema associated with amiodarone toxicity persists for several months. Serial measurements of the RNFL using OCT are essential in equivocal cases.
There are no formal recommendations for screening intervals, although a baseline exam is recommended by the Heart Rhythm Society (67). Given the typical onset within the first year of therapy, it has been suggested that a baseline exam, with interval exams during the first year, and annual exams thereafter is a reasonable approach (68). In patients who develop edema or vision changes, serial OCT of the RNFL may be helpful for following the resolution of edema and distinguishing between amiodarone-associated optic neuropathy and NAION. Improvement in vision was noted in 58% of patients after discontinuing amiodarone, so accurate diagnosis and consultation with the patient’s cardiologist is essential, particularly in more severe cases (67).
Linezolid toxicity
Linezolid is an oxazolidinone antibiotic that inhibits mitochondrial protein synthesis, and can cause optic neuropathy after prolonged use for greater than 1 month (69). Patients typically present with reduced visual acuity and color vision, with cecocentral scotomas and bilateral disc edema (70). Dramatic partial, if not complete, improvement is obtained with discontinuation of the medication. Temporal pallor is sometimes seen as a late finding. Only a small number of case reports have included OCT imaging, which shows diffuse RNFL thickening in the acute stage (70-72). Given the nonspecific OCT findings, a high level of suspicion in patients presenting with typical symptoms and prolonged use of linezolid (longer than 1 month) is essential for diagnosis. Prompt diagnosis is critical, as vision improves with withdrawal of linezolid. Steroid treatment has been attempted, but appears to worsen rather than improve symptoms (73,74).
Metabolic optic neuropathies
Deficiencies of cobalamin (vitamin B12), thiamine (vitamin B1), folate and copper have been associated with optic neuropathy (75-86). Symptoms are typical of mitochondrial optic neuropathy and include decreased visual acuity, dyschromatopsia and cecocentral scotoma. Patients often have an associated peripheral neuropathy, or macrocytic anemia in the setting of B12 or folate deficiency, but the optic neuropathy can occasionally be isolated. In the developed world, B12, thiamine and copper deficiency occur most often in the setting of poor absorption secondary to gastrointestinal disease or gastric bypass surgery. Folate deficiency has been associated with poor diet, alcoholism and long-term use of low-dose methotrexate. There have also been historical reports of outbreaks of optic neuropathy in populations suffering from nutritional strain due to sociopolitical factors, most recently the Cuban epidemic neuropathy from 1991–1993 (87).
The most comprehensive assessment of OCT findings in metabolic optic neuropathy is case series of 45 patients with B12 deficiency, which showed thinning of the average and temporal RNFL (88). Several case studies have been conducted on patients with copper deficiency who presented one to three years after gastric bypass surgery (75-78). Temporal pallor, with associated RNFL thinning was noted, along with GC/IPL thinning. The few case reports linking folate deficiency and thiamine deficiency with optic neuropathy have not included OCT, but one patient with folate deficiency did exhibit bilateral temporal disc edema (79-83). These data suggest that selective temporal thinning on OCT in the appropriate clinical context should raise concern for metabolic optic neuropathy.
Optic neuropathy in the setting of vitamin deficiency is often multifactorial. It is likely that the phenomenon referred to as “tobacco-alcohol amblyopia” results from a convergence of vitamin deficiency, the toxic effects of tobacco and alcohol, and genetic predisposition (89). Therefore, clinicians should be extremely cautious about rendering this diagnosis without a thorough investigation for other causes. Patients can present with multiple vitamin deficiencies concurrently, so it is often advisable to test comprehensively for B12, thiamine, folate and copper along with CBC to assess for macrocytic anemia. With the exception of copper, supplementation of the deficient vitamin typically results in excellent visual outcome.
Distinguishing mitochondrial optic neuropathies from glaucoma
Glaucoma is a common cause of optic neuropathy, with a global prevalence recently estimated at 3.54% in the population aged 40–80 years (90). The optic nerve head typically exhibits deep cupping without pallor until late in the course of disease. In contrast, mitochondrial optic neuropathies typically cause disc pallor within months of symptom onset with a variable amount of cupping. Glaucoma preferentially affects the superior and inferior poles of the optic nerve earliest, with corresponding arcuate visual field defects and sparing of central vision until late in the disease course. Therefore OCT demonstrating RNFL thinning superiorly, inferiorly and nasally is more suggestive of glaucoma, while isolated or more severe temporal thinning with macular GC-IPL thinning early is more characteristic of mitochondrial disease. However, OCT alone is not diagnostic, and the entire clinical picture must be taken into account. As toxic and metabolic optic neuropathies can be easily treated with improvement in visual outcomes, a thorough medication review is recommended and nutritional evaluation should be undertaken in the appropriate clinical context.
Distinguishing mitochondrial optic neuropathies from ischemia
Non-arteritic anterior ischemic optic neuropathy (NAION), resulting from microvascular disease, and arteritic anterior ischemic optic neuropathy (AAION), which occurs in the setting of giant cell arteritis (GCA), present with unilateral, acute onset disc edema, vision loss, dyschromatopsia and scotomas that typically respect the horizontal midline. Over the course of 6 to 8 weeks, the disc edema subsides, with the development of disc pallor that is diffuse, or restricted to the superior or inferior aspect of the nerve, with associated RNFL and GC-IPL thinning on OCT. As in glaucoma, the sectoral nature of optic atrophy can be helpful in distinguishing between ischemic optic neuropathies and mitochondrial disorders.
Distinguishing mitochondrial optic neuropathies from other causes of optic atrophy
Posterior to the lamina cribrosa, the optic nerve is myelinated and is subject to inflammatory demyelinating diseases including multiple sclerosis and neuromyelitis optica spectrum disorders (NMOSD). Infiltrative inflammatory or malignant processes including sarcoidosis, lymphoproliferative disorders, optic nerve sheath meningioma, and optic nerve gliomas can also involve the orbital or intracanalicular segments of the optic nerve, as can numerous infectious agents including lyme, syphilis and tuberculosis (91). Mass lesions within the orbit can cause a compressive optic neuropathy, and traumatic optic neuropathy results from shear or compressive forces incurred by the optic nerve as it travels through the restrictive space of the optic canal. These diverse etiologies tend to impact the full thickness of the optic nerve to cause decreased visual acuity and color vision, an afferent pupillary defect (APD) (if unilateral), and visual fields with generalized depression. There is sometimes disc edema in acute phases, with later progression to diffuse pallor, with RNFL and GC-IPL thinning on OCT, although sectoral changes have been reported in a subset of cases.
Mass lesions causing compression in the pre-chiasmatic segment of the optic nerve can cause a junctional scotoma, with central vision loss and diffuse disc pallor of the involved side, and a superotemporal scotoma on the contralateral side, while lesions compressing the chiasm classically result in bitemporal hemianopsia, and occasionally cause bilateral temporal pallor of the disc and RNFL thinning on OCT. As discussed above, these findings are seen in a minority of patients with mitochondrial optic neuropathies. Imaging is strongly recommended to rule out mass lesions in the setting of bitemporal or junctional field cuts, particularly those that respect the vertical meridian.
Conclusions
Hereditary, metabolic and toxic optic neuropathies likely result from mitochondrial dysfunction, which disproportionately affects the PMB to cause bilateral central vision loss and dyschromatopsias. Characteristically, OCT shows RNFL thinning that is most pronounced temporally, but this can be delayed in appearance. Concordant ganglion cell/inner plexiform layer (GC-IPL) analysis is particularly useful, as it can show early changes prior to RNFL thinning. Overall, OCT is a valuable tool that can assist in the diagnosis of mitochondrial optic neuropathies.
Acknowledgments
Funding: This work was supported by an unrestricted grant from the Research to Prevent Blindness to the Washington University Department of Ophthalmology and Visual Sciences, and by a Research Award from the Vitreoretinal Surgery Foundation (JE).
Footnote
Provenance and Peer Review: This article was commissioned by the Guest Editors (Fiona Costello and Steffen Hamann) for the series “The Use of OCT as a Biomarker in Neuro-ophthalmology” published in Annals of Eye Science. The article has undergone external peer review.
Conflicts of Interest: Both authors have completed the ICMJE uniform disclosure form (available at http://dx.doi.org/10.21037/aes.2020.02.04). The series “The Use of OCT as a Biomarker in Neuro-ophthalmology” was commissioned by the editorial office without any funding or sponsorship. The authors have no other conflicts of interest to declare.
Ethical Statement: The authors are accountable for all aspects of the work in ensuring that questions related to the accuracy or integrity of any part of the work are appropriately investigated and resolved.
Open Access Statement: This is an Open Access article distributed in accordance with the Creative Commons Attribution-NonCommercial-NoDerivs 4.0 International License (CC BY-NC-ND 4.0), which permits the non-commercial replication and distribution of the article with the strict proviso that no changes or edits are made and the original work is properly cited (including links to both the formal publication through the relevant DOI and the license). See: https://creativecommons.org/licenses/by-nc-nd/4.0/.
References
- Ogden TE, Miller RF. Studies of the optic nerve of the rhesus monkey: nerve fiber spectrum and physiological properties. Vision Res 1966;6:485-506. [Crossref] [PubMed]
- Pan BX, Ross-Cisneros FN, Carelli V, et al. Mathematically modeling the involvement of axons in Leber's hereditary optic neuropathy. Invest Ophthalmol Vis Sci 2012;53:7608-17. [Crossref] [PubMed]
- Potts AM, Hodges D, Shelman CB, et al. Morphology of the primate optic nerve. 3. Fiber characteristics of the foveal outflow. Invest Ophthalmol 1972;11:1004-16. [PubMed]
- Webb SV, Kaas JH. The sizes and distribution of ganglion cells in the retina of the owl monkey. Aotus trivirgatus. Vision Res 1976;16:1247-54. [Crossref] [PubMed]
- Holländer H, Makarov F, Stefani FH, et al. Evidence of constriction of optic nerve axons at the lamina cribrosa in the normotensive eye in humans and other mammals. Ophthalmic Res 1995;27:296-309. [Crossref] [PubMed]
- Balducci N, Cascavilla ML, Ciardella A, et al. Peripapillary vessel density changes in Leber's hereditary optic neuropathy: a new biomarker. Clin Exp Ophthalmol 2018;46:1055-62. [Crossref] [PubMed]
- Balducci N, Savini G, Cascavilla ML, et al. Macular nerve fibre and ganglion cell layer changes in acute Leber's hereditary optic neuropathy. Br J Ophthalmol 2016;100:1232-7. [Crossref] [PubMed]
- Martins A, Rodrigues TM, Soares M, et al. Peripapillary and macular morpho-vascular changes in patients with genetic or clinical diagnosis of autosomal dominant optic atrophy: a case-control study. Graefes Arch Clin Exp Ophthalmol 2019;257:1019-27. [Crossref] [PubMed]
- Fraser JA, Biousse V, Newman NJ. The neuro-ophthalmology of mitochondrial disease. Surv Ophthalmol 2010;55:299-334. [Crossref] [PubMed]
- Sadun AA, La Morgia C, Carelli V. Leber's Hereditary Optic Neuropathy. Curr Treat Options Neurol 2011;13:109-17. [Crossref] [PubMed]
- Savini G, Barboni P, Valentino ML, et al. Retinal nerve fiber layer evaluation by optical coherence tomography in unaffected carriers with Leber's hereditary optic neuropathy mutations. Ophthalmology 2005;112:127-31. [Crossref] [PubMed]
- Barboni P, Savini G, Valentino ML, et al. Retinal nerve fiber layer evaluation by optical coherence tomography in Leber's hereditary optic neuropathy. Ophthalmology 2005;112:120-6. [Crossref] [PubMed]
- Barboni P, Carbonelli M, Savini G, et al. Natural history of Leber's hereditary optic neuropathy: longitudinal analysis of the retinal nerve fiber layer by optical coherence tomography. Ophthalmology 2010;117:623-7. [Crossref] [PubMed]
- Zhang Y, Huang H, Wei S, et al. Characterization of macular thickness changes in Leber's hereditary optic neuropathy by optical coherence tomography. BMC Ophthalmol 2014;14:105. [Crossref] [PubMed]
- Gaier ED, Gittinger JW, Cestari DM, et al. Peripapillary Capillary Dilation in Leber Hereditary Optic Neuropathy Revealed by Optical Coherence Tomographic Angiography. JAMA Ophthalmol 2016;134:1332-4. [Crossref] [PubMed]
- Kousal B, Kolarova H, Meliska M, et al. Peripapillary microcirculation in Leber hereditary optic neuropathy. Acta Ophthalmol 2019;97:e71-6. [Crossref] [PubMed]
- Matsuzaki M, Hirami Y, Uyama H, et al. Optical coherence tomography angiography changes in radial peripapillary capillaries in Leber hereditary optic neuropathy. Am J Ophthalmol Case Rep 2018;9:51-5. [Crossref] [PubMed]
- Klopstock T, Yu-Wai-Man P, Dimitriadis K, et al. A randomized placebo-controlled trial of idebenone in Leber's hereditary optic neuropathy. Brain 2011;134:2677-86. [Crossref] [PubMed]
- Vignal C, Uretsky S, Fitoussi S, et al. Safety of rAAV2/2-ND4 Gene Therapy for Leber Hereditary Optic Neuropathy. Ophthalmology 2018;125:945-7. [Crossref] [PubMed]
- Toomes C, Marchbank NJ, Mackey DA, et al. Spectrum, frequency and penetrance of OPA1 mutations in dominant optic atrophy. Hum Mol Genet 2001;10:1369-78. [Crossref] [PubMed]
- Votruba M, Fitzke FW, Holder GE, et al. Clinical features in affected individuals from 21 pedigrees with dominant optic atrophy. Arch Ophthalmol 1998;116:351-8. [Crossref] [PubMed]
- Fournier AV, Damji KF, Epstein DL, et al. Disc excavation in dominant optic atrophy: differentiation from normal tension glaucoma. Ophthalmology 2001;108:1595-602. [Crossref] [PubMed]
- Votruba M, Thiselton D, Bhattacharya SS. Optic disc morphology of patients with OPA1 autosomal dominant optic atrophy. Br J Ophthalmol 2003;87:48-53. [Crossref] [PubMed]
- Skidd PM, Lessell S, Cestari DM. Autosomal dominant hereditary optic neuropathy (ADOA): a review of the genetics and clinical manifestations of ADOA and ADOA+. Semin Ophthalmol 2013;28:422-6. [Crossref] [PubMed]
- Kjer B, Eiberg H, Kjer P, et al. Dominant optic atrophy mapped to chromosome 3q region. II. Clinical and epidemiological aspects. Acta Ophthalmol Scand 1996;74:3-7. [Crossref] [PubMed]
- Yu-Wai-Man P, Griffiths PG, Burke A, et al. The prevalence and natural history of dominant optic atrophy due to OPA1 mutations. Ophthalmology 2010;117:1538-46, 1546.e1.
- Barboni P, Savini G, Cascavilla ML, et al. Early macular retinal ganglion cell loss in dominant optic atrophy: genotype-phenotype correlation. Am J Ophthalmol 2014;158:628-36.e3. [Crossref] [PubMed]
- Provis JM, van Driel D, Billson FA, et al. Development of the human retina: patterns of cell distribution and redistribution in the ganglion cell layer. J Comp Neurol 1985;233:429-51. [Crossref] [PubMed]
- Barboni P, Carbonelli M, Savini G, et al. OPA1 mutations associated with dominant optic atrophy influence optic nerve head size. Ophthalmology 2010;117:1547-53. [Crossref] [PubMed]
- Rönnbäck C, Milea D, Larsen M. Imaging of the macula indicates early completion of structural deficit in autosomal-dominant optic atrophy. Ophthalmology 2013;120:2672-7. [Crossref] [PubMed]
- Johnston PB, Gaster RN, Smith VC, et al. A clinicopathologic study of autosomal dominant optic atrophy. Am J Ophthalmol 1979;88:868-75. [Crossref] [PubMed]
- Kjer P, Jensen OA, Klinken L. Histopathology of eye, optic nerve and brain in a case of dominant optic atrophy. Acta Ophthalmol (Copenh) 1983;61:300-12. [Crossref] [PubMed]
- Barboni P, Savini G, Parisi V, et al. Retinal nerve fiber layer thickness in dominant optic atrophy measurements by optical coherence tomography and correlation with age. Ophthalmology 2011;118:2076-80. [Crossref] [PubMed]
- Milea D, Sander B, Wegener M, et al. Axonal loss occurs early in dominant optic atrophy. Acta Ophthalmol 2010;88:342-6. [Crossref] [PubMed]
- Yu-Wai-Man P, Bailie M, Atawan A, et al. Pattern of retinal ganglion cell loss in dominant optic atrophy due to OPA1 mutations. Eye (Lond) 2011;25:596-602. [Crossref] [PubMed]
- Kim TW, Hwang JM. Stratus OCT in dominant optic atrophy: features differentiating it from glaucoma. J Glaucoma 2007;16:655-8. [Crossref] [PubMed]
- Corajevic N, Larsen M, Ronnback C. Thickness mapping of individual retinal layers and sectors by Spectralis SD-OCT in Autosomal Dominant Optic Atrophy. Acta Ophthalmol 2018;96:251-6. [Crossref] [PubMed]
- Ito Y, Nakamura M, Yamakoshi T, et al. Reduction of inner retinal thickness in patients with autosomal dominant optic atrophy associated with OPA1 mutations. Invest Ophthalmol Vis Sci 2007;48:4079-86. [Crossref] [PubMed]
- Russo A, Delcassi L, Marchina E, et al. Correlation between visual acuity and OCT-measured retinal nerve fiber layer thickness in a family with ADOA and an OPA1 mutation. Ophthalmic Genet 2013;34:69-74. [Crossref] [PubMed]
- Balducci N, Ciardella A, Gattegna R, et al. Optical coherence tomography angiography of the peripapillary retina and optic nerve head in dominant optic atrophy. Mitochondrion 2017;36:60-5. [Crossref] [PubMed]
- Kozak SF, Inderlied CB, Hsu HY, et al. The role of copper on ethambutol's antimicrobial action and implications for ethambutol-induced optic neuropathy. Diagn Microbiol Infect Dis 1998;30:83-7. [Crossref] [PubMed]
- Leibold JE. The ocular toxicity of ethambutol and its relation to dose. Ann N Y Acad Sci 1966;135:904-9. [Crossref] [PubMed]
- Chen SC, Lin MC, Sheu SJ. Incidence and prognostic factor of ethambutol-related optic neuropathy: 10-year experience in southern Taiwan. Kaohsiung J Med Sci 2015;31:358-62. [Crossref] [PubMed]
- Kho RC, Al-Obailan M, Arnold AC. Bitemporal visual field defects in ethambutol-induced optic neuropathy. J Neuroophthalmol 2011;31:121-6. [Crossref] [PubMed]
- Zoumalan CI, Agarwal M, Sadun AA. Optical coherence tomography can measure axonal loss in patients with ethambutol-induced optic neuropathy. Graefes Arch Clin Exp Ophthalmol 2005;243:410-6. [Crossref] [PubMed]
- Chai SJ, Foroozan R. Decreased retinal nerve fibre layer thickness detected by optical coherence tomography in patients with ethambutol-induced optic neuropathy. Br J Ophthalmol 2007;91:895-7. [Crossref] [PubMed]
- Kim U, Hwang JM. Early stage ethambutol optic neuropathy: retinal nerve fiber layer and optical coherence tomography. Eur J Ophthalmol 2009;19:466-9. [Crossref] [PubMed]
- Kim YK, Hwang JM. Serial retinal nerve fiber layer changes in patients with toxic optic neuropathy associated with antituberculosis pharmacotherapy. J Ocul Pharmacol Ther 2009;25:531-5. [Crossref] [PubMed]
- Masvidal D, Parrish RK 2nd, Lam BL. Structural-functional dissociation in presumed ethambutol optic neuropathy. J Neuroophthalmol 2010;30:305-10. [Crossref] [PubMed]
- Peng CX, Zhang AD, Chen B, et al. Macular thickness as a predictor of loss of visual sensitivity in ethambutol-induced optic neuropathy. Neural Regen Res 2016;11:469-75. [Crossref] [PubMed]
- Lee JY, Choi JH, Park KA, et al. Ganglion Cell Layer and Inner Plexiform Layer as Predictors of Vision Recovery in Ethambutol-Induced Optic Neuropathy: A Longitudinal OCT Analysis. Invest Ophthalmol Vis Sci 2018;59:2104-9. [Crossref] [PubMed]
- Lee JY, Han J, Seo JG, et al. Diagnostic value of ganglion cell-inner plexiform layer for early detection of ethambutol-induced optic neuropathy. Br J Ophthalmol 2019;103:379-84. [Crossref] [PubMed]
- Vieira LM, Silva NF, Dias dos Santos AM, et al. Retinal Ganglion Cell Layer Analysis by Optical Coherence Tomography in Toxic and Nutritional Optic Neuropathy. J Neuroophthalmol 2015;35:242-5. [Crossref] [PubMed]
- Chamberlain PD, Sadaka A, Berry S, et al. Ethambutol optic neuropathy. Curr Opin Ophthalmol 2017;28:545-51. [Crossref] [PubMed]
- Gümüş A, Oner V. Follow up of retinal nerve fiber layer thickness with optic coherence tomography in patients receiving anti-tubercular treatment may reveal early optic neuropathy. Cutan Ocul Toxicol 2015;34:212-6. [Crossref] [PubMed]
- Kim KL, Park SP. Visual function test for early detection of ethambutol induced ocular toxicity at the subclinical level. Cutan Ocul Toxicol 2016;35:228-32. [Crossref] [PubMed]
- Menon V, Jain D, Saxena R, et al. Prospective evaluation of visual function for early detection of ethambutol toxicity. Br J Ophthalmol 2009;93:1251-4. [Crossref] [PubMed]
- Sharpe JA, Hostovsky M, Bilbao JM, et al. Methanol optic neuropathy: a histopathological study. Neurology 1982;32:1093-100. [Crossref] [PubMed]
- Liesivuori J, Savolainen H. Methanol and formic acid toxicity: biochemical mechanisms. Pharmacol Toxicol 1991;69:157-63. [Crossref] [PubMed]
- Desai T, Sudhalkar A, Vyas U, et al. Methanol poisoning: predictors of visual outcomes. JAMA Ophthalmol 2013;131:358-64. [Crossref] [PubMed]
- Nurieva O, Diblik P, Kuthan P, et al. Progressive Chronic Retinal Axonal Loss Following Acute Methanol-induced Optic Neuropathy: Four-Year Prospective Cohort Study. Am J Ophthalmol 2018;191:100-15. [Crossref] [PubMed]
- Zakharov S, Pelclova D, Diblik P, et al. Long-term visual damage after acute methanol poisonings: Longitudinal cross-sectional study in 50 patients. Clin Toxicol (Phila) 2015;53:884-92. [Crossref] [PubMed]
- Klein KA, Warren AK, Baumal CR, et al. Optical coherence tomography findings in methanol toxicity. Int J Retina Vitreous 2017;3:36. [Crossref] [PubMed]
- Abrishami M, Khalifeh M, Shoayb M, et al. Therapeutic effects of high-dose intravenous prednisolone in methanol-induced toxic optic neuropathy. J Ocul Pharmacol Ther 2011;27:261-3. [Crossref] [PubMed]
- Pakdel F, Sanjari MS, Naderi A, et al. Erythropoietin in Treatment of Methanol Optic Neuropathy. J Neuroophthalmol 2018;38:167-71. [Crossref] [PubMed]
- Mansour AM, Puklin JE, O'Grady R. Optic nerve ultrastructure following amiodarone therapy. J Clin Neuroophthalmol 1988;8:231-7. [PubMed]
- Passman RS, Bennett CL, Purpura JM, et al. Amiodarone-associated optic neuropathy: a critical review. Am J Med 2012;125:447-53. [Crossref] [PubMed]
- Johnson LN, Krohel GB, Thomas ER. The clinical spectrum of amiodarone-associated optic neuropathy. J Natl Med Assoc 2004;96:1477-91. [PubMed]
- De Vriese AS, Coster RV, Smet J, et al. Linezolid-induced inhibition of mitochondrial protein synthesis. Clin Infect Dis 2006;42:1111-7. [Crossref] [PubMed]
- Javaheri M, Khurana RN, O'Hearn TM, et al. Linezolid-induced optic neuropathy: a mitochondrial disorder? Br J Ophthalmol 2007;91:111-5. [Crossref] [PubMed]
- Ishii N, Kinouchi R, Inoue M, et al. Linezolid-induced optic neuropathy with a rare pathological change in the inner retina. Int Ophthalmol 2016;36:761-6. [Crossref] [PubMed]
- Park DH, Park TK, Ohn YH, et al. Linezolid induced retinopathy. Doc Ophthalmol 2015;131:237-44. [Crossref] [PubMed]
- Frippiat F, Bergiers C, Michel C, et al. Severe bilateral optic neuritis associated with prolonged linezolid therapy. J Antimicrob Chemother 2004;53:1114-5. [Crossref] [PubMed]
- Saijo T, Hayashi K, Yamada H, et al. Linezolid-induced optic neuropathy. Am J Ophthalmol 2005;139:1114-6. [Crossref] [PubMed]
- Naismith RT, Shepherd JB, Weihl CC, et al. Acute and bilateral blindness due to optic neuropathy associated with copper deficiency. Arch Neurol 2009;66:1025-7. [Crossref] [PubMed]
- Pineles SL, Wilson CA, Balcer LJ, et al. Combined optic neuropathy and myelopathy secondary to copper deficiency. Surv Ophthalmol 2010;55:386-92. [Crossref] [PubMed]
- Rapoport Y, Lavin PJ. Nutritional Optic Neuropathy Caused by Copper Deficiency After Bariatric Surgery. J Neuroophthalmol 2016;36:178-81. [Crossref] [PubMed]
- Shah AR, Tamhankar MA. Optic neuropathy associated with copper deficiency after gastric bypass surgery. Retin Cases Brief Rep 2014;8:73-6. [Crossref] [PubMed]
- Balachandran C, McCluskey PJ, Champion GD, et al. Methotrexate-induced optic neuropathy. Clin Exp Ophthalmol 2002;30:440-1. [Crossref] [PubMed]
- Clare G, Colley S, Kennett R, et al. Reversible optic neuropathy associated with low-dose methotrexate therapy. J Neuroophthalmol 2005;25:109-12. [Crossref] [PubMed]
- de Silva P, Jayamanne G, Bolton R. Folic acid deficiency optic neuropathy: a case report. J Med Case Rep 2008;2:299. [Crossref] [PubMed]
- Hsu CT, Miller NR, Wray ML. Optic neuropathy from folic acid deficiency without alcohol abuse. Ophthalmologica 2002;216:65-7. [Crossref] [PubMed]
- Spinazzi M, Angelini C, Patrini C. Subacute sensory ataxia and optic neuropathy with thiamine deficiency. Nat Rev Neurol 2010;6:288-93. [Crossref] [PubMed]
- Hamilton HE, Ellis PP, Sheets RF. Visual impairment due to optic neuropathy in pernicious anemia: report of case and review of the literature. Blood 1959;14:378-85. [Crossref] [PubMed]
- Longmuir R, Lee AG, Rouleau J. Visual loss due to Wernicke syndrome following gastric bypass. Semin Ophthalmol 2007;22:13-9. [Crossref] [PubMed]
- Surges R, Beck S, Niesen WD, et al. Sudden bilateral blindness in Wernicke's encephalopathy: case report and review of the literature. J Neurol Sci 2007;260:261-4. [Crossref] [PubMed]
- González-Quevedo A, Santiesteban-Freixas R, Eells JT, et al. Cuban Epidemic Neuropathy: Insights into the Toxic-Nutritional Hypothesis through International Collaboration. MEDICC Rev 2018;20:27-31. [PubMed]
- Türkyılmaz K, Oner V, Turkyilmaz AK, et al. Evaluation of peripapillary retinal nerve fiber layer thickness in patients with vitamin B12 deficiency using spectral domain optical coherence tomography. Curr Eye Res 2013;38:680-4. [Crossref] [PubMed]
- Carroll FD. Nutritional amblyopia. Arch Ophthalmol 1966;76:406-11. [Crossref] [PubMed]
- Tham YC, Li X, Wong TY, et al. Global prevalence of glaucoma and projections of glaucoma burden through 2040: a systematic review and meta-analysis. Ophthalmology 2014;121:2081-90. [Crossref] [PubMed]
- Kahloun R, Abroug N, Ksiaa I, et al. Infectious optic neuropathies: a clinical update. Eye Brain 2015;7:59-81. [PubMed]
Cite this article as: Enright J, Van Stavern G. Application of optical coherence tomography in hereditary, toxic and metabolic optic neuropathies. Ann Eye Sci 2020;5:17.