Multifunctional nanotherapeutics for treatment of ocular disease
The eye is an important organ, it provides vision and it is an important component of our facial identity (1,2). There is an old saying “The eye is the window of the soul”, clear and bright eyes bring esthetic pleasure to people. Human eye is globular and consists of two main parts, the anterior and posterior segments (1,2). Although the posterior part of the eye is comfortably located in the orbit, they are delicate because its anterior segment including the cornea is exposed to the outside world, thus accessible to wear and tear. To protect and maintain the eye functions while reduce the disruptions from outside and inside the body, the eyes are equipped with defense mechanisms for both the anterior and posterior segments (1,2). For the cornea region, the eyes are associated with precorneal factors including eyelids, tear film, blinking, tear turnover, induced lacrimation and nasolacrimal drainage. Cornea itself contains multilayers of corneal epithelial cells with tight junctions, which severely limit ocular penetration of molecules (3,4). For intraocular environment (mostly the posterior parts), it contains two main barriers, blood-aqueous and blood-retina barrier with epithelial and endothelial tight junctions, which restrict penetration of molecules into the intraocular chamber (5). All these features make the eye an isolated and well-protected organ, however, they also make it hard to treat during ocular diseases (1,2).
Eye infection is the most common eye disease, and serious infections including fungal keratitis could often cause ocular morbidity and blindness (1,2,6). Traditional therapy involves systemic administration of antibiotics including intravenous injection and oral dosing. However, the ocular barriers as mentioned before could hamper the penetration of majority of the drugs and only 1–2% of administrated drugs can gain access to the retina and vitreous body (1,2,6). Topical administration including eye drops and topical injections are also popular for eye treatments. Eye drops are the main form of topical administration due to good patient compliance and economic considerations. However, due to the corneal barrier and pre-corneal factors, less than 5% of the total administered drugs can penetrate the cornea and the residence time is less than 5 minutes (1,2,6). While intraocular injection is common, it is an invasive method that could cause a series of side effects (1,2,6).
To overcome these limitations, it is important to develop alternative therapeutics with increased bioavailability, prolonged retention time, and sustained drug delivery (1,2,6). The advances in nanotechnology are revolutionizing the medical field and new generation of therapeutics is making strides in the treatment of ocular diseases. Nanotechnology is a technology focusing on the nano-scale (size range, 1–100 nm), which is also the scale that biology operates on because most biological molecules including protein, DNA, RNA are nanometer in size (1,2,7). However, it is not just the scale that matters, for nanotechnology, it changes our thinking on how to solve a problem, in this case, design a new therapeutic. It breaks down the traditional disciplinary barriers and uses a multidisciplinary approach, including material science, chemistry, physics, engineering, biology, medicine, and whatever it takes, to solve a real-world problem, which is rarely solvable by one discipline (1,2,7). Nanotechnology is making inroads on the development of theranostics for ocular diseases (1,2). For example, nanostructured dendrimer-based hydrogels were shown to be effective in closing corneal laceration without suturing of the cornea and promote rapid wound healing without scar formation and inflammation (8). Gene therapy including gene knock-down by polymers carrying shRNA against VEGF could reduce the corneal neovascularization in vivo (9). For glaucoma diagnosis, flexible and implantable wireless micro-electro-mechanical systems (MEMS) nano-sensors are used to monitor intraocular pressure (IOP) (10,11). Nanostructures including polymers, dendrimers, liposomes, nanospheres and nanocapsules carrying drugs are used to increase the ocular residence time based their high affinity to the mucin layer on cornea (2,12,13). In addition, hydrogel-based contact lens has been developed as an effective drug delivery system to treat ocular diseases (2,6). This is best exemplified by the featured ACS Nano article by Yuan and Jiang (6) (Figure 1).
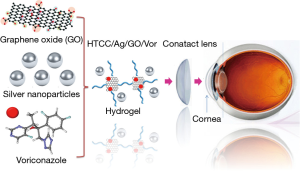
Contact lenses are successfully utilized for vision correction (1,2,6). The comfort level of their use is high and they have high capacity for drug loading that makes them suitable as an ocular drug delivery system (1). The last few decades have witnessed advances in hydrogel research and nanotechnology that enable soft contact lenses to carry and release drugs at sustained rates to achieve more efficient therapeutic effects with fewer side effects (1). Yuan and Jiang et al. designed and fabricated a hybrid hydrogel-based contact lens which comprises quaternized chitosan (HTCC), silver nanoparticles, and graphene oxide (GO) with a combination of antibacterial and antifungal functions (6). The hydrogel is cross-linked through electrostatic interactions between GO and HTCC (14,15), resulting in strong mechanical properties. Voriconazole (Vor), a hydrophobic antifungal drug, can be loaded onto GO via π-π stacking while retains the drug, which undergo sustained release from the hydrogel-based contact lenses (6). The contact lenses also exhibited good antimicrobial functions by introducing silver nanoparticles, which serve as a reservoir to continuously shed antimicrobial silver ions (16-19). It is worth noting that GO is also known to have antimicrobial capability by inducing membrane disruption via generation of reactive oxygen species (20). HTCC also shows antimicrobial effects likely due to the cationic functional groups that could induce membrane damage via electrostatic binding of negatively charged lipid bilayers (6,21-23). The results from in vitro and in vivo experiments demonstrate that contact lenses loaded with Vor and silver nanoparticles have excellent efficacy in antifungal activity on a fungus-infected mouse model (6). The results indicate that this hydrogel contact lenses-based drug delivery system might be a promising therapeutic approach for a rapid and effective treatment of fungal keratitis (6).
It is notable that a successful demonstration like this is not an easy task. It is not a simple compilation of different nano-components and expects an effective therapeutic outcome. To develop a contact lens based on hydrogel, one has to consider the mechanical properties such tensile strength and elasticity, water content, ion permeability, transparency, oxygen permeability, and toxicity (1,6). For example, the addition of GO to HTCC enhances the mechanical properties of the contact lens, while HTCC alone does not form intact lens (6). GO is also able to bind Vor and allow its sustained release, and GO itself has antimicrobial ability via direct contact with microorganisms (6,20). In addition, both HTCC and silver nanoparticle endow the contact lens with antimicrobial capability. Furthermore, it is important to make sure the safety of nanoformulations (24,25). The nanosystem is proven to be safe and nontoxic to the cornea demonstrating good biocompatibility (6). The seamless integration of these nanocomponents while maintaining the necessary properties to form the stable contact lens is the key to the success of the nanosystem (6). It is foreseeable that similar integrated approaches could be developed to treat other ocular diseases using nanotechnology.
Since the advent of nanotechnology many novel theranostics have been developed for ocular diseases including infection, glaucoma, neovascularization, and cancer (1,2,8-11,13). The therapeutic contact lenses are an excellent alternative to treat corneal diseases (1,2). Although there are many attempts in this platform, the commercialization of such products in the market is still limited (1,2). The issues often regard to the critical lens properties including transparency, mechanical property, water content, ion and oxygen permeability for comfortable wearing of contact lens day and night. Additional issues that concern the drug stability, zero order release kinetics without burst release, protein adherence, drug release during storage in packaging solution, shelf life, cost and benefit analysis, etc. have to be considered for medical applications (1,2). Only when these issues are addressed satisfactorily, the therapeutic contact lens can change the way that ocular diseases are treated (1).
Acknowledgments
Funding: This work was primarily supported by the National Institute of Environmental Health Sciences (NIEHS) R01 ES016746 and ES022698 as well as U01 ES027237, with leveraged support from the National Science Foundation (NSF) and the Environmental Protection Agency (EPA) under Cooperative Agreement Number DBI 0830117 and 1266377. Additional support is from “Hundred Talents Program” of Chinese Academy of Sciences and National Natural Science Foundation of China (31570899).
Footnote
Provenance and Peer Review: This article was commissioned and reviewed by Section Editor Jin Yuan, MD, PhD (State Key Laboratory of Ophthalmology, Zhongshan Ophthalmic Center, Sun Yat-sen University, Guangzhou, China).
Conflicts of Interest: The author has completed the ICMJE uniform disclosure form (available at http://dx.doi.org/10.21037/aes.2017.03.06). The author has no conflicts of interest to declare.
Ethical Statement: The author is accountable for all aspects of the work in ensuring that questions related to the accuracy or integrity of any part of the work are appropriately investigated and resolved.
Open Access Statement: This is an Open Access article distributed in accordance with the Creative Commons Attribution-NonCommercial-NoDerivs 4.0 International License (CC BY-NC-ND 4.0), which permits the non-commercial replication and distribution of the article with the strict proviso that no changes or edits are made and the original work is properly cited (including links to both the formal publication through the relevant DOI and the license). See: https://creativecommons.org/licenses/by-nc-nd/4.0/.
References
- Maulvi FA, Soni TG, Shah DO, et al. A review on therapeutic contact lenses for ocular drug delivery. Drug Deliv 2016;23:3017-26.
- Sharaf MG, Cetinel S, Heckler L, et al. Nanotechnology-Based Approaches for Ophthalmology Applications: Therapeutic and Diagnostic Strategies. Asia Pac J Ophthalmol (Phila) 2014;3:172-80.
- Dingeldein SA, Klyce SD. The topography of normal corneas. Arch Ophthalmol 1989;107:512-8.
- Prausnitz MR, Noonan JS. Permeability of cornea, sclera, and conjunctiva: a literature analysis for drug delivery to the eye. J Pharm Sci 1998;87:1479-88.
- Cunha-Vaz JG. The blood-ocular barriers: past, present, and future. Doc Ophthalmol 1997;93:149-57.
- Huang JF, Zhong J, Chen GP, et al. A Hydrogel-Based Hybrid Theranostic Contact Lens for Fungal Keratitis. ACS Nano 2016;10:6464-73.
- Nel AE, Mädler L, Velegol D, et al. Understanding biophysicochemical interactions at the nano-bio interface. Nat Mater 2009;8:543-57.
- Oelker AM, Berlin JA, Wathier M, et al. Synthesis and characterization of dendron cross-linked PEG hydrogels as corneal adhesives. Biomacromolecules 2011;12:1658-65.
- Gonzalez L, Loza RJ, Han KY, et al. Nanotechnology in corneal neovascularization therapy--a review. J Ocul Pharmacol Ther 2013;29:124-34.
- Piffaretti F, Barrettino D, Orsatti P, et al. Rollable and implantable intraocular pressure sensor for the continuous adaptive management of glaucoma. Conf Proc IEEE Eng Med Biol Soc 2013;2013:3198-201.
- Todani A, Behlau I, Fava MA, et al. Intraocular pressure measurement by radio wave telemetry. Invest Ophthalmol Vis Sci 2011;52:9573-80.
- Bravo-Osuna I, Noiray M, Briand E, et al. Interfacial interaction between transmembrane ocular mucins and adhesive polymers and dendrimers analyzed by surface plasmon resonance. Pharm Res 2012;29:2329-40.
- Khutoryanskiy VV. Advances in mucoadhesion and mucoadhesive polymers. Macromol Biosci 2011;11:748-64.
- Ji QX. In vitro evaluation of the biomedical properties of chitosan and quaternized chitosan for dental applications. Carbohydr Res 2009;344:1297-302.
- Yang Y, Wang J, Zhang J, et al. Exfoliated graphite oxide decorated by PDMAEMA chains and polymer particles. Langmuir 2009;25:11808-14.
- George S, Lin S, Ji Z, et al. Surface defects on plate-shaped silver nanoparticles contribute to its hazard potential in a fish gill cell line and zebrafish embryos. ACS Nano 2012;6:3745-59.
- Ma J, Liu R, Wang X, et al. Crucial Role of Lateral Size for Graphene Oxide in Activating Macrophages and Stimulating Pro-inflammatory Responses in Cells and Animals. ACS Nano 2015;9:10498-515.
- Wang Z, Xia T, Liu S. Mechanisms of nanosilver-induced toxicological effects: more attention should be paid to its sublethal effects. Nanoscale 2015;7:7470-81.
- Zhang H, Wang X, Wang M, et al. Mammalian Cells Exhibit a Range of Sensitivities to Silver Nanoparticles that are Partially Explicable by Variations in Antioxidant Defense and Metallothionein Expression. Small 2015;11:3797-805.
- Li R, Mansukhani ND, Guiney LM, et al. Identification and Optimization of Carbon Radicals on Hydrated Graphene Oxide for Ubiquitous Antibacterial Coatings. ACS Nano 2016;10:10966-80.
- Xia T, Kovochich M, Liong M, et al. Polyethyleneimine coating enhances the cellular uptake of mesoporous silica nanoparticles and allows safe delivery of siRNA and DNA constructs. ACS Nano 2009;3:3273-86.
- Xia T, Kovochich M, Nel AE. Impairment of mitochondrial function by particulate matter (PM) and their toxic components: implications for PM-induced cardiovascular and lung disease. Front Biosci 2007;12:1238-46.
- Li R, Wang X, Ji Z, et al. Surface charge and cellular processing of covalently functionalized multiwall carbon nanotubes determine pulmonary toxicity. ACS Nano 2013;7:2352-68.
- Nel A, Xia T, Mädler L, et al. Toxic potential of materials at the nanolevel. Science 2006;311:622-7.
- Xia T, Li N, Nel AE. Potential health impact of nanoparticles. Annu Rev Public Health 2009;30:137-50.
Cite this article as: Xia T. Multifunctional nanotherapeutics for treatment of ocular disease. Ann Eye Sci 2017;2:22.